Inheritance of Flower Colours And Breeding Of Evergreen Azaleas
Dr. J. Heursel
Institute of Ornamental Plant Growing
Melle, Belgium
1. Introduction
Since evergreen azaleas are vegetatively propagated through cuttings, it is possible to obtain one good F-1 plant from crossing two varieties, to multiply it and thus to create a new variety. This new variety can in turn be vegetatively propagated. Apart from mutations and sports it will always remain the same. This may sound very simple, but in reality, of course, it is not. It will be obvious that, because of the severe demands made on a new variety, it is extremely difficult to combine all the desired characteristics in one new azalea.
It is only through carrying out numerous crosses and through breeding a very large number of seedlings that success may be achieved. One good variety can be expected out of 10,000 seedlings. However, this seemingly low efficiency in breeding needs to be looked at in perspective; it is counter-balanced by the fact that the average age of the evergreen azalea cultivars is 37 years. A good new variety lasts for a long time. A more efficient approach towards breeding is possible only if the heredity of the many characteristics in which azaleas differ from one another is known.
Research on breeding can be of help here. It attempts to clear the way for the production of new varieties. Its aim is to gather, very gradually, all the basic information conducive to breeding.
A lot is already known about the inheritance of flower colours in azaleas. In order to gain a good understanding of this, some genetic terms will need to be explained.
Every plant consists of a very large number of cells. These contain a nucleus, in which are found a fixed number of chromosomes that can be made visible as small bars. In these chromosomes we find the genes. They are responsible for hereditary characteristics. A normal cell is diploid, which means that the chromosomes are divided into pairs. Every chromosome, and consequently also every gene, is twofold. The reason for this is the following: every new azalea is created from the fusion of an egg cell and a pollen grain. Before egg cell and pollen grain are ready for fusion, the reduction of the chromosome number from the paired condition (diploid) to the single (haploid) condition is necessary.
As a result of this the number of chromosomes equals n = 13. This is called the haploid number. Through the fusion of egg cell and pollen grain the number of chromosomes (n + n = 2n = 26) is restored to its original level. As a result of the initial reduction and the subsequent fusion it remains constant from generation to generation. With regard to the genes that are responsible for hereditary characteristics such as flower colour, two things can happen at the fusion of egg cell and pollen.
The new plant receives the same hereditary information from its father and from its mother. It receives, for instance, from both parents the gene red for the characteristic flower colour. Such a plant is called "homozygous" for red.
On the other hand, the new plant may have received red from one parent and white from the other. This plant, then, is called "heterozygous". We are now faced with the question: what colour will this plant be, if it inherits red from one parent and white from the other? A hierarchy exists among alleles. There is almost always one allele that dominates. This one is called dominant, the subordinate one recessive.
2. Inheritance Of Flower Colour On A Visual Basis
When we assess the inheritance of flower colours in azaleas on a visual basis or by means of a colour chart, the situation is as follows. We may divide azaleas into four groups: purple ('Adria', 'Blue Danube'); carmine red ('Vuyk's Scarlet'), red ('Stewartstonian', 'Rex') and white. Colour, whether it be purple, carmine red or red, is dominant over white. Red is dominant only over white. Carmine is dominant over red. Purple is dominant over carmine. Inversely, red is recessive to carmine, and carmine to purple. The order is, therefore, headed by purple followed by carmine red, red and white. A summary is contained in Table 1.
Table 1
- Summary of the inheritance of colours in evergreen azaleas
purple x purple = purple
carmine x purple = purple
carmine x carmine = carmine
red x purple = purple
red x carmine = carmine
red x red = red
white x colour = colour
white x white = white
Thus, for example, the cross 'Stewartstonian' x 'Rex' (red) will produce red progeny only, because red x red = red.
Anyone who regularly carries out crosses, will have found that in practice results other than those indicated in Table 1 are obtained. In order to make clear the basic principle, we have assumed that both parents are homozygous. Most cultivars, however, are heterozygous, because they are cross-pollinators. Homozygosity is obtained mainly through self-pollination. Let us now look at how the inheritance of white, red and carmine red occurs according to Mendel's laws. It is customary in genetics to represent a gene by an arbitrary letter from the alphabet. The dominant allele is represented by a capital letter, the recessive one by a small letter. In the simplest case at the bottom of Table 1, where the flower colour is determined by one single gene, we represent the gene "colour" by W, and white w is recessive.
We represent homozygous for red by WW, that is to say, the symbol W once for each parent from which the plant has sprung. Thus, white becomes ww. The cross of white and red produces:
white ww x red WW In this case the offspring is red Ww, because red is dominant over white. The offspring is, however, heterozygous Ww. This is in agreement with Mendel's first law. Let us now cross the descendants among themselves, as represented in the following scheme:
F-1 Ww x Ww
W w
W WW Ww
w Ww ww
F-2 1WW 2Ww 1ww
3red 1 white
Red x red now results in a three red and one white ratio, in accordance with Mendel's second law. Because azaleas are heterozygous, it is nearly always the last result that is obtained from a cross.
The same reasoning applies also to the inheritance of carmine red to red. In this case, however, there are two genes for the colour carmine red, i.e. W and Q. Let us represent carmine red by Q (dominant) and non-carmine red by q. Non-carmine red (q) can then be red, if W is present for colour in general, if not, white because no colour can be formed. Carmine (Qq) x carmine (Qq) will result in 3 carmine and 1 red, because both parents are heterozygous. An azalea heterozygous for colour and carmine (WwQq) crossed with a white wwqq will result in carmine (WQ), red (Wq) and 2/4 white (wQ and wq). This is clarified in the following scheme:
WQ wQ Wq wq
wq carmine white red white
3. Inheritance Of Pigments
Anthocyanins
When crossing with purple, it will be difficult to find the Mendelian ratios. The inheritance pattern discussed above, which is based exclusively on the external phenotypical observation of flower colours, does not allow us to explain why the white
R. mucronatum
('Ledifolia Alba') crossed with red flowers results in a purple progeny. This fact was observed by Wada (1968) and undoubtedly also by Joseph Gable.
It is necessary for this to know the heredity of the pigments that are responsible for the different flower colours. They were examined by De Loose (1970) and Van Sumere et al. (1985). Only if the pigments are known is it possible to study their heredity.
When the gene W is present in a dominant, although not necessarily homozygous, state, the red pigment cyanidin is produced (see Figure 1, left). Examples of cultivars containing cyanidin are: 'Addy Wery', 'Lysande', 'Mijnheer Oscar Wuyts' and 'Morgenzon'. Moreover, these four cultivars differ from one another in that the sugars linked with cyanidin are different. It is, however, not the nature of these sugars that influences flower colour, but their number (position 3- or 3, 5).
A gene P is responsible for the methylation of cyanidin in position 3' and 5' (see Figure 1, top left). Only position 3' may be methylated to obtain the red pigment peonidin. We shall come back later on to the role of P in position 5'. An example of a cultivar containing peonidin as main anthocyanin is 'President Kennedy'. Some amount of cyanidin is, however, retained in the flower. In most cultivars containing peonidin the amount of cyanidin is even greater than that of peonidin. Examples are: 'Koningin Wilhelmina', 'Salmon Hinomayo' and 'Santa Maria'. As to their influence on visible colour, it does not make much difference whether the pigments consist mainly of cyanidin or of peonidin.
Peonidin-varieties are very common among Kurume azaleas, as opposed to Belgian Indian hybrids. If the gene W is recessive (ww), there is no production of red pigment.
Flavonols
In the absence of red pigments the flowers are white, but flavonols, such as quercetin, azaleatin, myricetin and myricetin 5-methylether (also linked with sugars) may well be formed. A gene Q is responsible for the production of quercetin (see Figure 1). If Q is recessive qq, we no longer find any flavonols. An example is the white 'Alaska'. One gene O is responsible for the oxidation of position 5' (see Figure 1). The resulting flavonol is myricetin. The gene M can methylate quercetin as well as myricetin in position 5. This then produces respectively azaleatin and myricetin 5-methylether. If Q is dominant, but M and O are recessive mmoo, the flower will contain only quercetin. An example is the white 'Anna Maria'. If M is dominant, but O is recessive oo, the flower will contain azaleatin. M methylates quercetin, yielding azaleatin. A white cultivar containing azaleatin does not occur among Kurume hybrids, but it does among Belgian Indian azaleas. An example is the white 'Niobe'.
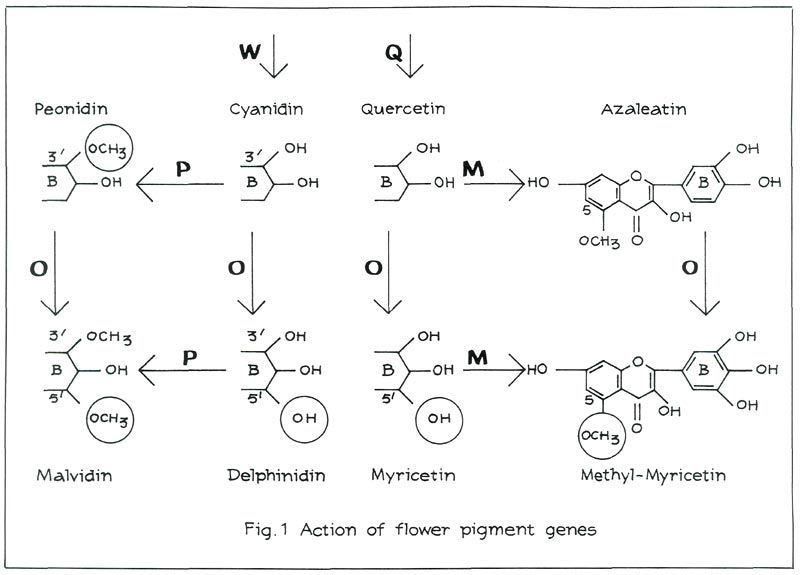
Inversely, no white cultivar containing quercetin was found among Belgian Indian azaleas. We have the impression that quercetin comes from the Kurume's and azaleatin from the Belgian Indian ones.
If Q is dominant, as well as M and O, myricetin 5-methylether will be produced. Examples are 'Adonis', 'Silbersee', 'White Lady' and
R. mucronatum
. White flowers containing flavonols can hardly be distinguished with the naked eye from white flowers not containing flavonols. In high concentrations, that is, from seven to tenfold, the flavonols not methylated in position 5 yield yellow flowers, such as with
Rhododendron keiskei
Miq. and
Rhododendron lutescens
Franch. Quercetin-3-galactoside contributes most to the yellowing in these cultivars, as in the case of
Rhododendron keiskei
.
As we have seen earlier, the gene P controls the 3'-5'-methylation. Position 5' can, however, be methylated only if it is oxidised. The gene O is responsible for this. We have already come across that gene in the case of the flavonols. It oxidises position 5' in the case of the anthocyanins as well as in that of the flavonols.
In the presence of gene O, the O.P. combination produces malvidin, which is responsible for purple flowers. 'Adria' and 'Blue Danube' are cultivars that contain malvidin (see Figure 1).
If P is recessive pp, but O is dominant, delphinidin is obtained. Plants containing delphinidin can be produced through crossing. The flower colours that range from purple to carmine are usually less pleasing. It is probably for this reason that there are no delphinidin varieties in the assortment. The
R. simsii
-cultivar 'Adonia' contains a large amount of delphinidin. If both O and P are recessive oopp, flowers containing cyanidin are produced.
Anthocyanins and flavonols combined
If the flavonols occur together with anthocyanins, there is a special bluing effect, which is called co-pigmentation. Carmine red is in effect produced by the addition of flavonols to the red pigments cyanidin or peonidin. 'Vuyk's Scarlet' contains the same anthocyanins as 'Adle', but the bluing effect is caused by the addition of flavonols (quercetin-glycosides).
R. mucronatum
is white (wwQQ OO PP), but carrier of the genes O and P. Crossed with the carmine red 'Hexe', for example, which contains the genes WWQqppoo, the combination W.Q.O.P. produces purple flowers containing malvidin, W.Q.O.P. being necessary for malvidin. It has now been made clear how in certain cases white x carmine red produces purple. For further details see Heursel and Horn 1979.
4. Flower Colour And Inheritance Of The Hose-In-Hose Character
From numerous crosses with hose-in-hose azaleas such as 'Hexe' and 'Kirin' the following results have occurred. Crosses with parents having green calyces always produce progeny with green calyces. Crosses of a mother plant having a green calyx (homozygous) with a father plant with a hose-in-hose calyx (heterozygous) produce 50 per cent hose-in-hose and 50 per cent green calyces. On the other hand, crossing a mother plant having a hose-in-hose calyx with a father plant having a green calyx almost never produces any progeny. Hose-in-hose x hose-in-hose, too, was unsuccessful. The reason for this is so far unknown. We may conclude that the green calyx is recessive to the hose-in-hose calyx. Let us represent the recessive green calyx by h and the hose-in-hose calyx by H.
As a result of this, no homozygous HH hose-in-hose plants occur. The cross of a green calyx hh x hose-in-hose Hh explains the 1:1 ratio green calyx: hose-in-hose.
Moreover, the hose-in-hose character and quercetin are sited on the same chromosome. They are linked to each other (see Figure 2 left). When there is linkage as in the case of 'Hexe' (carmine red
R. simsii
-cultivar containing quercetin), the cross carmine red, hose-in-hose x red, green calyx will again produce carmine red, hose-in-hose and red, green calyx. Carmine red, hose-in-hose and red, green calyx behave as one single characteristic because they are linked.
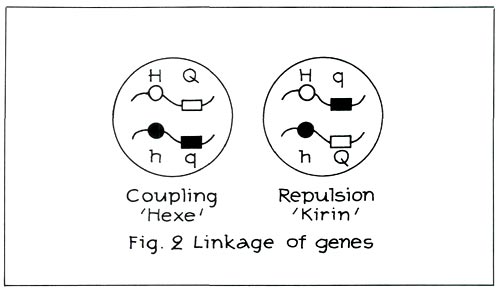
Nature will nevertheless see to it that this coupling does not occur in certain, admittedly rare, cases. This is, for instance, the case with 'Cangallo' and 'Kirin' ('Coral Bells'). This is called repulsion (see Figure 2, right). From this figure it can be deduced that the same genes are still present. They have, however, been re-ordered. The hose-in-hose character is no longer linked with quercetin, although quercetin is still present recessively.
When there is repulsion as in the case of 'Kirin', the cross carmine red, hose-in-hose x red, green calyx will now yield red, hose-in-hose and carmine red, green calyx! Examples of red hose-in-hose are: 'Blaauw's Pink' and 'Rex'.
5. Breeding A Yellow Evergreen Azalea
The first question that poses itself with regard to breeding of a yellow evergreen azalea is the following: where can we find the yellow pigments? There are two possibilities. The first yellow pigment is to be found in deciduous azaleas. They contain carotenoids, which produce strikingly beautiful shades of yellow and orange.
As this group is so well-known, it was obviously the first to be used. We have pollinated hundreds of flowers. In a few cases seed was obtained. However, virtually all the seedlings died during the first year through lack of chlorophyll. At one time a few intermediary seedlings were obtained that did not have any practical value because of slow growth. Since no carotenoids were found as flower pigments in evergreen azaleas, we are of the opinion that the chemical compositions of evergreen azaleas and those of deciduous ones are so widely different that the chances of successful crosses are too small.
A second yellow pigment are the flavonols present in the yellow
R. keiskei
and in
R. lutescens
. There is a very close chemical affinity with flavonols in evergreen azaleas.
Numerous crosses have been carried out. Again, anything can happen. The pollination may be unsuccessful, the seed capsule may suddenly fail to continue growing, after having developed initially. The seedlings may grow very badly and die after a year or remain vegetative for years. However, we also obtained offspring that developed in a normal way. From the cross 'Hinodegiri' x
R. keiskei
sprang real intermediaries, which are, however, completely sterile.
When offspring is produced, the result is usually dependent on the mother plant. If the mother plant is
R. simsii
, the descendants are pseudo-bastards resembling
R. simsii
-cultivars. Thus we obtained pseudo-bastards resembling
R. simsii
from the evergreen azalea 'Dame Blanche' (white 'Dame Melanie'-sport) as mother, and
R. lutescens
as father. With
R. lutescens
as mother, on the other hand, pseudo-bastards resembling
R. lutescens
were produced.
Preil and Ebbinghaus (1985), who crossed numerous
R. simsii
-cultivars as mother with
R. macabeanum
,
R. mollyanum
and
R. sinogrande
as father, also obtained pseudo-bastards resembling the mother. Motzkau (1982) has analogous results as well. As yet we do not have a satisfactory explanation for the phenomenon. In order to gain a deeper insight into this problem, we are engaged in cytological research on the embryo sac. It will take some time before we can produce a yellow evergreen azalea. This new difficulty contains at the same time new possibilities for further research. Even if it does not immediately produce the yellow azalea, it will lead us to new insights into breeding.
Another possibility that applies to deciduous azaleas as well as to those with a high flavonol content is an increase in the ploidy level of both crossing partners. This does not prove to be easy. The project offers perspectives that extend beyond the yellow azalea. Breeders of
Rhododendron
could benefit from this in the long term.
6. Gaining A Blue Evergreen Azalea
According to Asen et al. (1973) a blue flower colour is only to be expected from delphinidin co-pigmented with flavonols, combined with epidermal-tissue pH above 4. Because all evergreen azalea cultivars reveal a pH of the flower epidermal peel much lower than 4, the prospects for obtaining a blue flower colour are very small (De Loose 1978).
The first step in our research could consist of a chromatographic analysis of blue rhododendron flowers. This would deepen our insights into the way in which blue colour originates. If the evergreen azaleas turned out to be different, then maybe they would indicate an alternative way to blue. In any case, the way to blue seems to me even longer and more difficult than the one to yellow. Rhododendrons will continue to fascinate us into the next century. A large amount of research still needs to be done, before we can expect yellow or blue flowers by way of the latest discoveries in genetic manipulation. For the time being it seems to be the only possibility as far as blue is concerned.
Literature
De Loose, R., 1970. Flavonoid glycosides in the petals of some Rhododendron species and hybrids.
Phytochemistry
, 9: 875:879.
De Loose, R., 1978. Azalea indica flower colour as related to the parameters pH, anthocyanins and flavonol co-pigments.
Scientia Horticulturae
, 9: 285-290.
Heursel, J., 1975. Inheritance of the Flavonols Azaleatin and Quercetin in
Rhododendron simsii
Planch, and
Rh. obtusum
Planch.
Z. Pflanzenzchtg
. 74, 62-70.
Heursel, J., 1976. Die Vererbung des Merkmals "hose in hose" (Doppelkronigkeit) bij Azaleen.
Gartenwelt
, 76: 111-113.
Heursel, J., and W. Horn, 1977. A hypothesis on the inheritance of flower colours and flavonols in
Rhododendron simsii
Planch.
Z Pflanzenzchtg
. 79, 238-249
Motzkau, H., 1982. Personal communication.
Preil, W., and R. Ebbinghaus, 1985. Bastardier-ungen von Topfazaleen (
Rhododendron simsii
) mit anderen Rhododendron Arten. Lecture 7 Internationales Azaleen Seminar, Lehr- und Versuchsanstalt fr Gartenbau, D-2903-Bad Zwischenahn.
Van Sumere, C., Van De Casteele, K., De Loose, R., and J. Heursel. Reversed phase-HPLC Analysis of fiavonoids and the Biochemical Identification of cultivars of evergreen azalea. In:
Annual Proceedings of the Phytochemical Society of Europe Vol. 25, Biochemistry of plant phenolics
, 1985. Eds. C.F. Van Sumere and P.J. Lea Oxford University Press.
Wada, K., 1967. Rhododendron 'Mucronatum'. In:
The Rhododendron and Camellia Year Book
7968.
Dr. Heursel presented this paper at the Breeder's Roundtable session, ARS Annual Meeting, Cleveland, Ohio, May 1986.