JOTS v24n1 - An International Overview of Curricular Approaches and Models in Technology Education
An International Overview of Curricular Approaches and Models in Technology Education
Paul Black
Technology is a peculiar subject in that its status and its nature have been subject to radical changes in recent years. The subject is seen to serve several aims, which are given different relative priorities in different countries, and there are many traditions that are associated with competing pressures in the re-definitions of the subject. These changes and varieties are further complicated by the different curriculum models within which a reformed subject is meant to fit and play a specific role. It is suggested that the tensions between instrumentalist and humanist models for the subject may be dissolving, but that there are deeper problems about the nature of the learning involved in the fields of practical application. However, the most intractable problem is to implement very new pedagogy when the teaching force may be ill-prepared and where the classroom experience needed to temper and transform novel plans is lacking. PB
Dr. Black is Emeritus Professor in the School of Education at King's College in London, United Kingdom.
Technology is a constantly evolving subject, which is of great importance to the world's future. As it evolves, it is emerging from confusion, as the following quotations may illustrate. The first is from the recent New
Zealand formulation for their national curriculum:
Technology is a creative, purposeful activity aimed at meeting needs and opportunities through the development of products, systems or environments. Knowledge, skills and resources are combined to help solve practical problems. Technological practice takes place within, and is influenced by, social contexts. ( Ministry of Education, 1995, p. 6 )
This looks rather similar to the following:
. . . the area of human experience, skill and understanding that reflects man's concern with the material culture and with making and doing; that is with the appreciation and adaptation of his surroundings in the light of his material and spiritual needs. ( Roberts, 1994, p. 172 )
However, the latter is taken from a statement about design used in an article that argues that in curriculum formulation, it is design which should be central and technology subservient.
A further quotation is different again:
Productive work done by the pupils of grades 7 and 8 in earlier socialist industrial or agricultural enterprises was of a differentiated character, but it was generally concentrated in the same fields of work, and took place in "pupils production units," in enterprises in an "atmosphere of production," that is, under conditions of production adapted to the age of the pupils. ( Blandow & Mosna, 1994, p. 94 )
The idea of teaching technology was based on the belief that the production process is the source of social wealth and a purposeful pedagogical means of developing the individual's full personality. ( Blandow & Mosna, 1994, p. 97 )
Such differences arise in part from different traditions. Examples are the folk craft tradition, emphasizing making as a part of the cultural tradition, as in Sweden; technology seen as the practice of factory production in the older model of Eastern European socialism; or the movements in North America to treat technology as the application of science to be incorporated into a new area of study entitled science technology and society (STS). Often these different traditions have been promoted by competing interest groups, each claiming technology education as its own territory.
Many studies show that there are wide differences in the ways in which countries treat this area within compulsory schooling, although it will also be argued later in this paper that there are now signs of convergence. While it is recognized that it is of great importance, both for providing the basis of skills and of motivation for later vocational training and for giving balance to the education of the average citizen, there is uncertainty in many countries about how to define and establish the place of this important work in the school curriculum. McCormick (1991) discussed four main purposes for developing technology education, namely:
- Economic importance;
- Entrinsic value;
- Citizenship; and
- Marxist philosophy (now being abandoned in Eastern Europe).
There exist in the literature accounts from various countries of their school work in technology education (see for example Hacker et al., 1991 ; Layton 1994 ). These are in the main collections of descriptions of policy and practice. McCormick (1993) reported on the activities and motivation of the interest groups that have tried to influence technology education in the United Kingdom. A more ambitious study was attempted by the Organization for Economic Co-operation and Development (OECD) in its project on Innovation and Change in Science, Mathematics, and Technology Education. This was based on detailed case studies. However, the participating OECD countries offered far fewer studies in technology education than in science and mathematics ( Black & Atkin, 1996 ).
DIFFERENT APPROACHES TO TECHNOLOGY EDUCATION The list that follows (adapted and extended from de Vries, 1994 ) illustrates several different approaches, each of which may be underpinned by one or more of the above purposes:
- A technical skills approach, seeking emphasis on craft skills in treating resistant materials, food, and textiles, or in electronics and automatic control (e.g., Finland);
- A craft approach, in which the cultural and personal value of the combination of manual skill, aesthetic sensibility, and traditional design is to be preserved (e.g., the Swedish tradition of "Sloyd"; see Kananoja, 1994);
- A technical production approach, seeking emphasis on skills appropriate to modern mass production and its control and organization (Eastern Europe former socialist traditions);
- An engineering apprentice approach, seeing the school subject as a preparation ground for specialist technicians and engineers in tertiary education;
- A "modern technology" approach, which looks to the nature of "work" in the next century and focuses strongly on information technology (some even interpret the word technology to mean computers a strong tendency in the French approach);
- A "science and technology" approach in which it is assumed that these two subjects are, or ought to be, studied in close association with each other (as in Denmark; see Colding-Joergensen et al., 1990 );
- Concentration on design, seen by some as a central concept in the study and practice of technology (Northern Ireland);
- A problem-solving emphasis, focusing on an understanding of the nature of social needs in the definition of "problems" and of the need for a cross-disciplinary approach to tackling issues (Scotland, United States);
- A "practical capability" approach, emphasizing personal and active involvement of pupils in tackling realistic problems to offset the passive and receptive ethos of most of school education; and
- Emphasis on the technology-society nexus, which calls for study of technological innovation as a driving force for social change and of its interaction with other forces that also drive change (the STS movement).
Policy in some countries is mainly driven by one of these approaches, whereas in others there is an attempt to adopt several of them in concert. While some of them are closely matched to an emphasis on technology as a component of general education for all, others are linked to an emphasis on vocational preparation; on the other hand, assuming this emphasis is dominant, there can still be arguments over which of them gives the best preparation for employment needs.
The existence of this wide range of differences makes it difficult to communicate in discussions between and among countries. The school subject can carry a variety of names. For example, in the U.K. the terms technology , design and technology , technology and design , and craft design and technology have all been used, and each signifies a different rationale. The differences are often associated with struggles between competing interests to exert their influences on the school curriculum. Lack of consensus can inhibit policy development; thus, in the United States, groups have been in operation for several years to draw up and achieve consensus on statements of national standards in most of the main school subjects, but in technology a group to pursue this task was one of the last to be set up and, at the time of writing, has yet to report.
Curriculum ModelsEach of the approaches sketched above has to be implemented within a particular curriculum model. There are many possible overlaps between any school subjects that are or claim to be "technology" and other school subjects in related areas. Examples of such subjects are art and design, home care and home economics, business studies, "science through applications," craft skill training, and studies of business and industry. Thus, for example, studies of textiles and food feature in the U.K. curriculum for design and technology, but are located in a different subject area in the new Netherlands curriculum. More fundamentally, these different outcomes probably arise from different origins and assumptions about curriculum structures that underlie the partition between discrete subjects. Thus one curriculum philosophy can work from a concept of "areas of experience" and regard technology as a unique subject in covering one broad area; in such a case, the definition of the subject is driven by the need to play a particular role in an overall model of educational purposes. In other countries, the approach can be more pragmatic, regarding subjects as self-evidently necessary and having only a pragmatic philosophy for determining overlap disputes between different "subjects" or for developing links that would enhance the overall approach. The U.K. national curriculum is an example of this approach. The issues here may well be resolved only at the school level, so that it is the philosophy and management of the school as a unit that might be critical. To take the arguments further, it is helpful to look in detail at a few examples.
In Denmark, technology is not a separate subject, but is integrated as a component in the subjects "nature/technology" at the primary level (Grades 1-6) and physics/chemistry at the secondary level (Grades 7-9) ( Colding-Joergensen et al., 1990 ). It is taken by all pupils throughout, since there is no streaming according to abilities or to any other criteria and pupils stay together for the entire nine years in the Folkeskole. At the primary level, the same teacher often teaches across the curriculum. The curriculum guidelines emphasize objects from daily life and industrial production, including the interaction of technological development with nature and the conditions of human life. At the secondary level, the environmental impact of technological development is emphasized in biology and geography.
In Greece, there has been a gradual development since a technology component in the lower secondary school was provided for in legislation in 1976. The initial model emphasized a "practical-capability" approach, and pilot development has been influenced by developments in the state of Maryland, leading to adoption of a model with emphasis on the link between technology and society. However, it was only in 1993 that a decision was made to implement the model in all schools, and by 1995 the subject was offered in 280 out of a possible 1,800 schools. As the implementation expands, the problem of who should teach has become a particularly contentious one because with much teacher unemployment, different groups are pressing the Ministry to assign the subject to them.
In the Netherlands, technology was introduced in 1993 as one of 15 compulsory subjects for all pupils in the lower secondary school (12- to 14-year-olds). The implementation was preceded by a two-year part-time retraining program for teachers, coming from a variety of backgrounds, to be prepared to take responsibility for the new subject. The government also provided schools with funds to re-equip technology laboratories. The new subject replaces earlier teaching based on craft skills with wood, metals, and plastics. Now, textiles are added as a new material, but there is a more profound shift towards the learning of technological concepts and to covering some of the newer advanced technologies. The innovation was based on extensive debate about the broad aims. One of the influential statements specifies these as follows:
Education in Technology is aimed at familiarising ( sic ) the pupils with those aspects of Technology which are significant to the proper understanding of culture, to the way in which pupils function in society and [which are] significant to the pupils' further technical development. The pupils acquire knowledge and an understanding in the three main pillars of Technology (matter, energy and information), in the close relationship between Technology and the natural Sciences and between Technology and society. The pupils also learn how to produce Technology, i.e. "to become actively involved." ( Franssen, Eijkelhof, Duijmelinck, & Houtveen, 1995, p. 71 )
This vision, in which the practical activity is only a component of a broader plan, is a very ambitious and demanding one. Not surprisingly, some teachers resisted the broader aims of the new curriculum. They believed that pupils were attracted by the practical aspects of technology and could not cope with more theoretical reflection about the nature and the influences of technological activity. The commercially produced texts are varied, in that some have taken on the new concept-oriented and design-focused approach, while others have tried to link and incorporate new content into the previous "general techniques" approach. The evidence is that in the first years, teachers are finding it very hard to break away from giving closely specified and rather constrained tasks to pupils. It has been hard for them to develop appropriate student activities in the new regime, mainly because of problems of classroom control, of ensuring safety, and of providing the wide ranges of equipment and materials needed if pupils are to be free to pursue their own ideas ( Black & Atkin, 1996 ).
In England and Wales, there was a developing technology curriculum over the past 25 years with a variety of forms of the subject (e.g., Craft Design and Technology, or Control Technology) moving away from long-established work on making set-piece artifacts to develop skills in fashioning wood and metal. A new unified subject was created in 1990 under the new national curriculum for all pupils who are 5 to 16 years of age ( Black, 1991 ). The unification in the plans meant that former teachers of craft, design, and technology and of home economics (dealing with wood and textiles) and teachers of business studies and of art and design had to come together to implement a new subject ( Paechter, 1993, 1995b ; McCormick, 1993 ). The curriculum was very ambitious, and the early implementations were uneven. There was much criticism, particularly from professional engineers who feared that the broad range and the early emphases on social needs and on discussing the nature of technology would weaken the teaching of skills of design and construction. The curriculum has been revised, and it is now narrower in scope, with a clear emphasis on designing and making and with the comprehensive statement of aims in relation to technology and society all removed (Department of Education, 1995). All pupils have to work in both nonresistant materials (textiles and food) and resistant materials (wood, metal, and plastics) up to age 14, after which there is more flexibility. All have to study and work with control systems (electrical, electronic, mechanical, pneumatic) and structures. The tasks in which students are to be engaged are to include open tasks involving designing and making of products, focused practical tasks set up to develop particular knowledge and skills, and study of existing artifacts by testing, disassembling, and evaluating them. Although science and technology teachers were developing collaboration prior to the new curriculum and technology was even seen as part of the science curriculum in some schools, these links have been significantly weakened because both sets of teachers have been overburdened with the demands of the new curriculum.
In Australia, the Australian Education Council (AEC) in 1993 characterized the field as follows: "Technology is often used as a generic term to include all the technologies people develop and use in their lives. It involves the purposeful application of knowledge, experience and resources to create products and processes to meet human needs" (p. 3). Technology was designated as a key learning area, specified in four strands to include Designing, Making, and Appraising; Information; Materials; and Systems. Technology unifies the areas of materials, design and technology, design graphics, food and textiles, keyboarding, information technology, media studies, applied power technology, agriculture, CAD, and electronics.
The guidelines for the subject suggest that students should:
- Build on their experiences, interests, and aspirations in technology;
- Find and use a variety of technological information and ideas;
- Show how ideas and practices in technology are conceived;
- Explain technical languages and conventions;
- Take responsibility for designs, decisions, actions, and assessments;
- Trial their proposals and plans;
- Take risks when exploring new ideas and practices; and
- Be open-minded and show respect for individual differences when responding to technological challenges.
The Australian work emphasizes a "Design, Make, and Appraise" model of teaching technology (and science). This model involves students working from a project design brief to design an object or solution to a problem. As in the United Kingdom, the new approaches are being implemented at the same time as changes in the curricula of other subjects, notably mathematics and science.
In the recent proposals for the new curriculum in New Zealand, the broad vision in the definition of technology quoted at the beginning of this paper is followed by a complementary statement about technology education:
Technology education is a planned process designed to develop students' competence and confidence in understanding and using existing technologies and in creating solutions to technological problems. It contributes to the intellectual and practical development of students, as individuals and as informed members of a technological society. ( Ministry of Education, 1995, p. 7 )
This statement is then expanded in relation to educational, personal, cultural, environmental, and economic aims. Of particular interest is the statement about benefits educationally:
Educationally, students are motivated to participate in purposeful activities, enabling them to apply and integrate their knowledge and skills from many learning areas in real and practical ways. ( Ministry of Education, 1995, p. 7 )
The main targets for the curriculum are set out in three main strands:
- Technological knowledge and understanding;
- Technological capability; and
- Technology and society.
These are then spelled out in some detail in eight levels to reflect progression in learning in these three strands. It is also emphasized that activities should cover a range of nine contexts (such as home, community, business, and industry) and a range of seven areas of technology (biotechnology, electronics and control, food, information and communication, materials, production and process, and structures and mechanisms). There is also strong emphasis on project work, supported by many examples. The overall framework is to be achieved through the interweaving of the strands, the contexts, and the areas. A list of skills is specified, and links with the learning areas of languages, mathematics, science, social sciences, the arts, and health are all envisaged in the discussion. It is envisaged that technology will be part of the curriculum throughout all years 1 to 13 of compulsory schooling.
A particularly interesting feature is that four different models of implementation are offered:
- A separate time-tabled subject taught by its own teachers;
- A study integrating units or modules of technology taught by teachers from a range of disciplines;
- A combination of the first two options; and
- Suspension of the normal timetable so that a year group can focus solely on technology work.
Schools are to choose their own methods, the only requirement being that technology is taught in substantial sections and not dissipated across the curriculum.
ISSUES AND PERSPECTIVES These six examples illustrate a variety of issues. The example of Denmark is unique in locating work on technology within other subject areas. The New Zealand example goes further than the others in encouraging schools to adopt a cross-curricular model for the implementation of technology. Approaches of the types sketched out there seem to reflect the approach called for by Black and Harrison (1985) and exemplified in accounts of work in U.K. schools before their national curriculum ( Murray, 1990 ; Paechter, 1995a ). In such approaches, technology would differ in its character from traditional school subjects, in calling on such subjectsseen as analyticto provide resources for the necessary holistic task of tackling real problems.
There is some convergence between the different examples in that most reflect recent changes that have broadened the scope and educational ambition of technology. There are, of course, differences in detail between, say, the emphases and structure of the plan for Australia, for the Netherlands, and for New Zealand. The examples also illustrate some of the problems of radical and ambitious change. The timescale in Greece may seem very long, but the U.K. example and, to a smaller extent, the Netherlands one, all indicate the great difficulty of implementing such ambitions in classroom practice, calling as they do for new and very demanding ways of organizing the work of students.
There is a host of other issues that could be explored within these innovations. Examples are gender issues, the relationship between science education and technology education, the design perspective, assessment, implementation strategies, the role of information technology, and research in technology education.
Among these and numerous other issues that arise, I shall concentrate on three which seem to me to stand out. The first issue is that this "subject" stands at a peculiar point of tension in the struggle between instrumental and humanist views of education. While these two argue for the importance of technology education for quite different reasons, on deeper examination the differences between them start to dissolve.
For the instrumental view, a review of the research literature ( Olson & Henning-Hansen, 1994 ) brings out the shifts now occurring as it becomes clear that narrowly focused training is likely to serve only the short-term interests for today's jobs. Thus, national strategies are now moving towards emphasis on an education that develops broadly applicable skills or competencies, variously embellished as "generic" or "core." Pursuit of these raises many problems, particularly about whether the capacity to transfer such competencies across contexts can be learned. The movement, however, brings the instrumentalist project closer to a concentration on the common skills that all citizens need for many aspects of their lives.
Likewise, the humanist view shifts its boundaries as it confronts the possibility that technology has a distinctive place in our culture which education ought to reflect. One tradition holds that design, reflecting humankind's activity as maker and doer, deserves equal place with language and mathematics as a core component of education (Roberts, 1994). The frequently invoked aim to develop "practical capability" speaks for a school subject which is far from "academic" in the traditional sense, but which rather strives to bring together the experience of practical action with the development and use of the resources of knowledge and skill that other school subjects may supply. Such a view moves so close to the modern version of instrumentalism that a rapprochement seems feasible.
A second issue is that even if the above arguments could lead to a consensus, there would be serious problems about the nature of practical learning that the subject would have to pursue. The notable example here is exposed by Olson and Henning-Hansen (1994) who quoted Layton's (1991) own analysis of the problematic nature of teaching the application of science. It is hard to define what level or mode of knowing science is useful for practical applications. If this issue is looked at from the point of practice in the world, then it is clear that the developing world bears the scars of many naively impractical applications. By contrast, Appleton and Ilkkaracan (1994) quoted examples of the technological capabilities to innovate and adapt shown by women in several developing countries who adapt traditional knowledge through trial and error to solve problems about the supply and preparation of food that are vital to their survival.
Looked at in the light of learning theory, this issue is also problematic. On the one hand, the prospect that technology project work can develop practical capability, and so bring out potential strengths of students that present schooling does not tap, is an exciting one. On the other hand, there are thorny problems in achieving the transfer of learning that is required if cross-curricular approaches are to work. It is easy to make the task here sound easy by covering differences through use of a common descriptor, notably by saying that it's all "problem solving," but such rhetorical devices cannot bear close scrutiny ( McCormick et al., 1993 ). Indeed, the nature of the cognitive activities that students go through in technology work, involving linking knowledge to a formulation of solutions to complex problems, is not well understood and calls for some difficult research.
A third issue is the possibility of resolving the disagreements between the different interest groups within a broad humanist view. Any such resolution would involve a more broad and ambitious definition of technology education than any country has dared to develop. However, the protagonists would be the only people available to teach the subject, so unless their hearts and minds could subscribe to the new concept, little could happen. Here the issue of how to implement change arises. As Posch (1994) has argued, a central diktat is no solution. Not only does it offend the professionals and risk obstruction, it cannot possibly work because the implementation of any strategy has to be local, embedded in the particular classrooms in relation to local environments, industries, and so on. Thus, if the challenge of its fractured nature could be resolved, technology education would present a further challenge to the models and strategies for curriculum innovation that countries might wish to use.
References Australian Education Council. (1993). National statements and curriculum profiles . Canberra: Australian Education Council.
Appleton, H., & Ilkkaracan, I. (1994) . The technological capabilities of women and girls in developing countries. In D. Layton (Ed.), Innovations in science and technology education: Vol. 5 (pp. 145158). Paris: UNESCO.
Black, P. J., & Harrison, G. B. (1985) . In place of confusion: Technology and science in the school curriculum. London: Nuffield-Chelsea Curriculum Trust and the National Centre for School Technology, Trent Polytechnic.
Black, P. J., & Harrison, G. B. (1992) . Pour Sortir de la Confusion: Technologie et Sciences dans les Curricula. In R. Levrat (Ed.), Technologie: Textes de Reference (pp. 87-131). Paris: Centre International D'Etudes Pedagogiques.
Black, P. J. (1991) . Technology education in the national curriculum for England and Wales. In M. Hacker, A. Gordon, & M. de Vries (Eds.), Integrating advanced technology into technology education (NATO ASI Series Vol. F78, pp. 167178). Heidelberg, Germany: Springer-Verlag.
Black, P. J., & Atkin, J. M. (1996) . Changing the subject: Innovations in science, mathematics and technology education. London: Routledge.
Blandow, D., & Mosna, F. (1994) . Technology education in Central and Eastern Europe. In D. Layton (Ed.), Innovations in science and technology education: Vol. 5 (pp. 91102). Paris: UNESCO.
Colding-JoergensenM , P., Jensen, J. H., Joergensen, R. C., Paulsen, A. C., & Paulsen, E. (1990). Teknologi og samfund som en dimension i fysikundervisningen. Copenhagen: Direktoratcy for Gymnasieskolerne of Hf.
Department of Education. (1995). Design and technology in the national curriculum . London: Her Majesty's Stationery Office.
de Vries, M. (1994) . Technology education in Western Europe. In D. Layton (Ed.), Innovations in science and technology education: Vol. 5 (pp. 3144). Paris: UNESCO.
Franssen , H. A. M., Eijkelhof, H. M C., Duijmelinck, H. A. J. P., & Houtveen, A. A. M. (1995). An in-depth study of technology as a school subject in junior secondary schools in the Netherlands. Utrecht, Netherlands: Department of Education, Utrecht University.
Hacker, M., Gordon , A., & de Vries, M. (Eds.). (1991). Integrating advanced technology into technology education (NATO ASI Series Vol. F78). Heidelberg, Germany: Springer-Verlag.
Kananoja, T. (1994) . Technology education in the Nordic countries. In D. Layton (Ed.), Innovations in science and technology education: Vol. 5 (pp. 4558). Paris: UNESCO.
Layton, D. (1991) . Science education and praxis: The relationship of school science to practical action. Studies in Science Education , 19, 4379.
Layton, D. (Ed.). (1994) . Innovations in science and technology education: Vol. 5. Paris: UNESCO.
McCormick, R. (1991) . The evolution of current practice in technology education. In M. Hacker, A. Gordon, & M. de Vries (Eds.), Integrating advanced technology into technology education (NATO ASI Series Vol. F78, pp. 4155). Heidelberg, Germany: Springer-Verlag.
McCormick, R. (1993) . The coming of technology education in England and Wales. In F. Banks (Ed.), Teaching technology (pp. 4755). London: Routledge.
McCormick, R., Hennessy, S., & Murphy, P. (1993) . The myth of general problem-solving capability: Design and technology as an example. The Curriculum Journal, 4(1) , 7389.
Ministry of Education, New Zealand . (1995). Technology in the New Zealand curriculum. Wellington, New Zealand: Learning Media.
Murray, R. (Ed.). (1990) . Managing design and technology in the national curriculum. London: Heinemann Educational.
Olsen, J., & Henning-Hansen, J. (1994) . Research on technology education. In D. Layton (Ed.), Innovations in science and technology education: Vol. 5 (pp. 225239). Paris: UNESCO.
Paechter, C. F. (1993) . What happens when a school subject undergoes a sudden change of status? Curriculum Studies , 1, 349364.
Paechter, C. F. (1995a) . Crossing subject boundaries: The micropolitics of curriculum innovation. London: Her Majesty's Stationery Office.
Paechter, C. F. (1995b) . Subcultural retreat: Negotiating the design and technology curriculum. British Educational Research Journal , 21 , 7587.
Posch, P. (1994) . Strategies for the implementation of technology education. In D. Layton (Ed.), Innovations in science and technology education: Vol. 5 (pp. 201212). Paris: UNESCO.
Roberts, P. (1994) . The place of design in technology education. In D. Layton (Ed.), Innovations in science and technology education: Vol. 5 (pp. 171179). Paris: UNESCO.
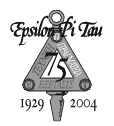

TS