JOTS v24n1 - Delivering Content to Achieve Industrial Technologist Performance Behaviors
IDEAS
Delivering Content to Achieve Industrial Technologist Performance Behaviors
by John W. Sinn
While this article can stand alone, the reader will be better served to also read the two companion pieces that preceded this one in previous journal issues. They are "Building a Model for Technology Studies," Volume 22, Number 2, Summer/Fall 1996, and "Universal Performance Behaviors: What Ought to Be in the Technologist's Toolkit," Volume 23, Number 1, Summer/Fall 1997. The first article described the model and the underlying rationale that guided its development. The second in the series articulated and identified behaviors that ought to be part of the array of competencies, or behaviors, of all technologists, regardless of their specific professional assignment. The body of thought presented in these three articles has been influenced by the author's work as director of the Center for Quality, Measurement, and Automation (CQMA) in the College of Technology at Bowling Green (Ohio) State University. CQMA's experiences with a number of technology transfer projects for business and industrial clients have contributed much to the refinement of the ideas presented here. Also, participation in the development of an Applied Quality Science bachelor's degree program further tested, refined, and demonstrated the efficacy of the model as did more recent experiences with a research project conducted for the American Society for Quality Control to define core knowledge for technologists. Details and reports of these experiences may be obtained from the author.
JS
The preceding note refers to the previously discussed model of technology and the behaviors of technologists. These lead to this brief discussion about the content and the organization of instruction thought best to prepare students and trainers to function as effective technologists. The models and the behaviors can be said to comprise a "toolkit" that technologists draw upon in their professional work. This third element, content and organization of instruction, tells us how that toolkit can be outfitted.
CORE KNOWLEDGE The term core knowledge is used in preference to the term content . Core knowledge is demonstrated in technologist behaviors that combine appropriate cognitions and appropriate process actions. Thus it is fitting to mix content with active process to educate and train technologists. Five core areas are derived from the model and the technologists' functional behaviors: (a) primer tools, (b) cultural tools, (c) data tools, (d) documentation tools, and (e) synchronous leader tools.
Primer tools introduce and provide an overview of the total technological system. The intent is to provide understanding of the broader system. Problem solving and team building are also introduced. As the name suggests, primer tools should be used in introductory-level courses as a foundation for all other content and knowledge. The logic of the primer tools is to provide the context for micro and macro functions that could occur in various ways within technological studies. There are 12 primer knowledge tools:
- Technology systems and industrial technology introduced.
- Teams, problem solving, change, and improvement in industrial technology and systems.
- Technical foundations for industry and technology.
- Materials and processes.
- Process engineering, design, and innovation.
- Automation and computer integration.
- Maintenance and safety.
- Quality systems.
- Service and training.
- Cost analysis and productivity improvement.
- Technology and management.
- Industrial technology and the future: Local, organizational, and global cultural issues.
Cultural tools are based on the primer tools and provide basic cultural definitions. They deal with the total system for world class organizational functions, technical empowerment, and change. They enable students to understand such things as why we are changing as related to broader market forces and the nature of internal and external environmental issues, among other macro circumstances. These tools form the basis for a robust and rigorous general education course that focuses on issues such as defining technology within the context of organizational mission and philosophy, organizational values and culture, characteristics of technological behavior, global resources, environment and development, technological diversity, and management and decision making as related to technology. There are 12 cultural tools:
- Technological organization and culture: Introduction to the toolkit.
- Evolution of the technological culture: Significant developments and values introduced.
- Characteristics of the technological culture: Kaizen change system for improvement.
- Critical technical and innovative underpinnings: Understanding product and process.
- Quality and productivity issues: Understanding and serving the customer.
- Managing, decision making, and control in the technological culture.
- Data-based improvement and solutions for change.
- Documentation for quality and productivity improvement: Kaizen foundations.
- Technological change, empowerment, and the learning organization.
- Political correctness and ethical issues.
- Environmental and resource issues.
- Soul of Technology: technology transfer, global development, and ongoing improvement.
Data tools focus on data for improvement and provide basic statistical definitions and an orientation to the total system of technical problem solving and improvement. The basic statistical concepts that should be addressed focus on gathering and assuring integrity in data. A first technical course in quality or statistics can bring to bear many of the issues essential for foundations of total quality and international standards. As with other tools, data tools provide incremental focus on building teams for technical problem solving by introducing and applying standard deviation, variable and attribute charting systems, sampling, repeatability and reproducibility, capability, measurement systems, and geometric dimensioning and tolerancing. There are 12 data tools:
- Team skills, organization, and data-driven technical problem solving.
- Brainstorming, root causes and effects, and identifying the problem.
- Basic definitions for data-based improvement, and foundations for improvement.
- Attribute data, histograms, bar and pareto charting: The obvious starting point.
- Variable data, comparisons to attribute charting, and short-run systems introduced.
- Metrology and onspection systems: Basic measurement issues.
- Gage repeatability and reproducibility (R&R), inspection, and measurement improvement.
- Capability analysis, interpreting out-of-control conditions, and SPC in transition.
- Quality characteristics, data-based actions, and systems documentation for ongoing improvement.
- Advanced measurement concepts and systems: Geometric and coordinate considerations.
- 8-D disciplined approach to data and systems-based documentation and problem solving.
- Design of experiments introduced: Enhanced team processes for improvement.
Documentation tools build on basic data and cultural concepts and enable technologists to use basic tools for analysis and problem solving. Systematic approaches to analysis of information and documentation are presented and pursued and are focused on continuous improvement techniques. Derived in part from the previous data set of tools, a broader framework for supplier and customer communication is provided. Tools in this group include costs, flow charting, process documentation, standard procedures, quality plans, failure mode analysis, quality function deployment, mistake proofing, and others. There are 12 documentation tools designed specifically for assessment functions:
- Total quality, world class, and productivity: Foundational Kaizen definitions and functions.
- Basic economic considerations for Kaizen quality and improvement.
- Advanced team skills and approaches: Making Kaizen meetings and teams work.
- Synchronous techniques: Just in time, Kaizen improvement further defined.
- Ongoing process control plan (OPCP): Kaizen systems for improvement.
- Understanding our process: Kaizen foundations.
- Standard operating procedures (SOP): Documentation for Kaizen improvement.
- Failsafing, mistakeproofing, enhanced design, innovation, and the five whys.
- The Kaizen service function: Understanding customer and supplier relationships.
- Benchmarking, evaluation, and measuring our successes.
- Robust Kaizen documentation for problem solving: QFD and FMEA.
- Future documentation: ISO and other concurrent systems considerations for problem solving.
Synchronous leader tools lead to the ultimate of technologist performance. These focus the previous tools more fully toward team-based technical problem solving and leadership and are particularly concerned with infrastruc-tural issues for ongoing improvement in the organization and of people. This set of tools can make up a course that capstones the previous tools. They are intended to present and tie together a myriad of broad issues over the longer term. They focus on how to grow talent for the organization, enable new product developments, and ensure robust quality and appropriate technical support systems. Significant issues to be addressed include how to lead in building systems for using data and documentation in problem-solving teams, synchronizing the myriad of vital functions organizationally and technologically for getting product out day to day in a managed fashion, and planning, developing and re-engineering the organizational culture and talent to meet future demands for competition. There are 12 synchronous leader tools:
- Ongoing improvement, synchronized change: Leadership and implementation.
- ISO and QS 9000, quality and launch systems: Broad relationships for our synchronous future.
- Leadership and supervision for the future: Synchronized quality planning and improvement.
- Enhanced design processes, innovation, and applied research for product development.
- Technical material and process considerations for product improvement and development.
- Synchronized data systems for quality planning and management.
- Advanced product and quality problem solving: Documentation systems synchronized.
- Automation and quality relationships: Data acquisition and communication systems.
- Maintenance functions and total productive maintenance: Maintainability and reliability.
- Safety, ergonomics, and human factors: Improved quality and productivity work environment.
- Growing talent, knowledgable workers, and the learning organization.
- Design of experiments, finite element analysis: Robust problem solving.
Organizing Teaching and Learning
These 60 content tools comprise useful knowledge that can be drawn upon in constructing learning experiences to achieve technologist behaviors. This can happen when they properly are commingled with relevant teaching and learning techniques. Put another way, whenever possible, instruction and training should be structured to provide settings, activities, processes, and situations that closely parallel those that occur in the practicing technologists' environment.
Problem solving and team building ought to permeate the learning process whenever possible. Planning for both long- and short-term functions, knowledge of product and process, and abilities to work at different levels and on teams in cross-functional ways reflect types of technical management issues. Instructors of technology subjects should seek to integrate these elements that are related to professional practice in their courses. In this way the core knowledge will be acquired in a context that carefully replicates professional activities. When so integrated, course or training outcomes in the form of student performance behaviors can be more easily achieved. This is demonstrated in the remainder of this article wherein selected elements of core knowledge are discussed along with teaching/learning modes and critical work contexts that require complex behavioral interactions.
The Technical Cultures
The technical culture can be achieved in organizational training programs through indoctrination courses for new employees or in training programs for senior employees. For example, when explaining internal or external customer or supplier functions, emphases could focus on values, civility, political and social characteristics, and other "core" issues.
A recently published case study that describes the use of the 12 data tools for training provides an example of knowledge of cultural issues required for building the technical culture. During the training in data-based tools, it became clear that various cultural issues had to be attended to, including the following:
- Assisting in developing and nurturing the growth and evolution of teams. This included everything from identifying and writing procedures to actually showing people how to do the procedures.
- Reinforcing cultural changes associated with empowermentmost people really did not believe that they could/should do things on their own. This is simply a self-confidence issue in many respects.
- Establishing standard procedures for conduct and protocol for meetings. Simple rules had to be identified and established.
- Writing standard procedures for collection and use of data, helping to provide the discipline in people to actually do the task correctly, and assuring that variation in process could be seen.
This demonstrated the significance of cultural issues and the reader should be aware that as the changes occurred in learning to use the actual tools, technical infrastructural changes also needed to occur in the culture of the organization ( Barker & Sinn, 1997 ).
Other content also addresses and explains technological functions. In the curriculum this could happen within the technical concentration and may also be acquired in business studies and other areas that provide understanding of how we function and survive in a competitive global marketplace. In the school setting the preceding also occurs under the general education component.
In both training or school settings, students should have reflective experiences that encourage creative thought, diversity, openness to change and flexibility, and other already identified core values. In all of these venues trainees and students should have regular opportunities to enhance communication skills and perform presentation functions that assist in defining culture and reinforce the technical concentration.
Data, Documentation, and Synchronous Leader
Data, documentation, and synchronous leader content is connected to technical teams and are fundamental tools in producing technical solutions and managing change and improvement. The myriad of data and documentation tools are requisite to adequately conduct analysis and assessment functions and for successful problem solving for improvement. While these should be taught directly, they also may be applied in other technical courses and at the team level. Significantly, when documentation and data are managed properly and synchronized with all other functions, they provide the basis for effective leadership and change.
For example, we have found that, it was only after gathering and applying a significant amount of properly documented data that the solution could be brought forward in a meaningful and productive manner for an actual product application for improvement. This also tells us that making critical design improvements for production quality while getting product out the door requires a maturity rooted in data, documentation, and synchronous leader experience.
Team-Based Empowerment and Cross-Functional Change
An empowered environment does not just happen by chance. It must be carefully designed and implemented. It should be based on a strong philosophy and a technological infrastructure. This core knowledge is oriented to change and education. The major impetus behind empowerment is education about the tools required to solve technical problems to achieve quality and productivity improvement. This drives technological change. Here, the technologist is the essential leader for the future, a technological change agent who is critical to cultural competitiveness. Instruction and training would provide for learning in self-management situations, individual and team evaluations, and continuous learning for improvement. These can be structured in such courses and experiences as applied business, the social and hard sciences, mathematics, and statistics.
An exciting elaboration of the team-based empowerment and cross-functional change principles and tools comes from a course taught by this author. It includes the following:
- Teams of students linked with mentors and projects on campus for internal infrastructural improvement on real projects at the university.
- Multiphased reports on the status of the projects and applications of the tools are provided and placed on the web.
- Much of the course and cross-functional teamwork is accomplished virtually electronically with no paper and decreasing amounts of "physical" meetings (traditional classroom time and teams).
Projects have ranged from building better university recruitment systems to defining and analyzing departmental quality systems and from analyzing and improving residence hall services to ordering, storing, and handling of materials purchased for campus operations.
A second example is contained in an undergraduate Total Quality Systems course and a graduate Quality and Reliability course, in which graduate and undergraduate teams are innovatively linked to cross function for actual product improvement. Recently, students have focused on the manufacture of aluminum triangles. Teams have designed, tested, modified, and produced approximately 35 triangles over the past several years. Interestingly, these have been used by the "customer," Epsilon Pi Tau, in its initiations around the world. Full-blown production of these products occurs in all respects. An ongoing enterprise environment is fully physically simulated, but under control for academic study. All aspects of change and empowerment, with a shared product and laboratory, are explored by graduate/undergraduate teams, including a focus on technical support, quality, and production deliverables as separate entities but with shared cross functions. While the teams do have shared meetings, as with cross-functional teams in any organization, they also meet as groups in separate graduate and undergraduate circumstances. Pivotal in the process is the distinction of leadership and management occurring at the graduate level, with graduate students mentoring undergraduates while working together toward shared objectives.
Finally, real projects for industrial firms provide instructional/learning experiences. Usually they are built around all of the technologist behavior attributes. Industrial projects, like much that has already been described, have grown out of industrial advisory committee activities over the past several years. A recent project exemplifies the approach, which was conducted at a manufacturing facility located near Bowling Green State University and was focused on improving the operation of a stamping press. Students met on site (rather than at the university) each week during the entire semester. Student teams were mentored by the manufacturing manager who also supplied essential facilities and information to support, nurture, and grow the team.
To be successful, the teaching/learning situations must exemplify those elements that parallel technologist behaviors. Thus, innovative and change-adaptive approaches help. For example, team teaching between faculty and industrial persons in courses taught at industrial sites for undergraduate and graduate students can be successful. These applied technical problems and projects were arranged and pursued. Other approaches include linking graduate students as team leaders with undergraduates on projects and placing nontraditional empowered teams in traditional curricula, similar to what some industrial firms do. These are not easy-to-administer approaches, but because they are effective learning situations, they are worth the effort ( Sinn, 1996 ; Waggoner, Rex, & Sinn, 1993 ).
In two preceding articles, a conceptual model of technology and the derived behaviors common to all technologist practice were presented. In this article, the content and organization of learning and instruction to accomplish these behaviors is reviewed. These three comprise a set of guidelines for training courses in industry and core studies in college or university technology curricula.
Three questions were addressed to help elaborate on the model and introduce the technologists' toolkit:
- What are universal technologists' behaviors?
- What core content, or tools, should be in the technologists' toolkit?
- How should teaching be organized and how may learning experiences be structured to best achieve technologist behaviors?
Just as the technologist ought never leave well enough alone, this article raises the following questions:
- Is there additional content that should be identified as core knowledge?
- Do data, documentation, and synchronous leader tools help explain critical knowledge for all technologists?
- Should such content be organized and taught in the form of tools?
- In much the same way that the 60 content tools speak to re-engineering and changing organizations, might the technologists' toolkit actually be core knowledge that, if adapted widely, can lead to a positive transformation of technological studies?
- Might considerations such as in these articles establish the technologists' rightful place and purpose in our culture?
References Barker, W., & Sinn, J. W. (1997) . Tools for technologists: A case study in team-based technical problem solving. Journal of Industrial Technology, 13 (4), 3-8.
Sinn, J. W. (1996) . Concurrent engineering in action: Getting started on the future . mfg.: The Brown & Sharp Measuring Systems Group, 3 (1), 48-49.
Waggoner, T., Rex, N., & Sinn, J. W. (1993) . Development of an off-campus 2+2 manufacturing program: Case study of an innovative educational program. The Journal of Technology Studies, 23 (1), 20-25.
Waggoner, T. , Trucker, C., Sinn, J. W., Huang, Z., & He, Y. (1995). Finite element analysis (FEA) in design and production. The Journal of Technology Studies, 21 (2), 20-27.
Material on courses mentioned in this article may be viewed at web site: http://www.bgsu.edu/colleges/technology/Manufac/CQMA/302/ .
Once at the web site, actually pulling down the project presentations and classwork requires installation and use of acrobat reader software (freely available as a "click-on" once at the site).
Dr. Sinn is a Professor and Director of the Center for Quality, Measurement, and Automation in the College of Technology at Bowling Green State University, Ohio. He is a Laureate member of Alpha Gamma Chapter of Epsilon Pi Tau.
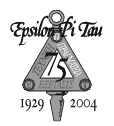

TS