JOTS v35n1 - Nanotechnology Education: Contemporary Content and Approaches
Nanotechnology Education: Contemporary Content and Approaches
Jeremy V. Ernst
Abstract
Nanotechnology is a multidisciplinary field of research and development identified as a major priority in the United States. Progress in science and engineering at the nanoscale is critical for national security, prosperity of the economy, and enhancement of the quality of life. It is anticipated that nanotechnology will be a major transitional force that possesses the potential to change society. Rapid and continued advancement in the field of nanotechnology is accelerating the demand for specific professional knowledge and skill. These lines of technological discovery and improvement continue to unlock new content for classroom incorporation.
Contemporary approaches and practices to further engage learners and enhance their abilities to apply nanoscale-related content knowledge must be continually developed in order for the United States to solidify itself as the primary builder of nanotechnology research and development. Steadfast development of new technologies leading to continual transformation of society serves as a strong indicator that current educational practices should be altered in order to prepare knowledgeable and engaged citizens. The use of three-dimensional graphics, virtual reality, virtual modeling, visualizations, and other information and communication technologies can assist in reinforcing nano-associated scientific and technological concepts.
Introduction
High-level content of pressing importance addressed through contemporary approaches is an identified necessity in educational systems. Development and innovation are critical components of education in the United States; they promote the maturity of an educated, skilled, and creative general public. Emerging technologies, such as medical technologies, biotechnologies, and especially nanotechnologies represent prosperous areas for the workforce and the economy, and therefore, they can be a driving force in career and technical education. According to trend analysis, the future will be largely dominated by amplified use of nanotechnologies ( The National Academies, 2006 ). Longitudinal analysis leaves little uncertainty that nanotechnology will guide a key transformation with a significant impact on society ( Mnyusiwalla, Daar, & Singer, 2003 ).
Although there has been a steady growth in global nanotechnology, this dominating trend is especially true for the United States. The National Academies (2006) indicates that 33 percent of all nanotechnology patents awarded from 1990 to 2004 were granted to researchers in the United States. Japan was second, with 19 percent of the worldwide patents during the same period of time.
Shelley (2006) indicates that determined research and development for targeted nanoscience and nanotechnology has resulted in discovery and application now culminating in marketable products and new commercial applications. As more products (e.g., sunscreens, cosmetics, clothing, upholstery, paint, bodywork of vehicles, computer components) utilize nanotechnologies, it is only a matter of time until nanotechnology infiltrates consumer products holistically. Shelley (2006) further identifies the limitless possibilities that applying nanotechnology presents. He asserts that the span and reach of nanotechnology should become and remain a primary consideration not only in government and commerce but also in education. This consideration stems from nanotechnology’s application to energy, medical, and information technology, which are both essential and developing components of today’s society. The purpose of this article is to introduce nanotechnology/nanotechnology education, its societal and economic significance and its importance as an area of study. Also provided are examples and recommendations on the implementation of nanotechnology into teacher-preparation programs.
Literature Review
The National Aeronautics and Space Administration (as cited in Mnyusiwalla, Daar, & Singer, 2003 ) identifies the field of nanotechnology as focusing on the formation of purposeful materials, devices, and systems through the management of matter on the nanometer scale, and the utilization of new occurrences and attributes at the scale. Lakhtakia (2006) indicates that nanotechnology is not a solitary method; neither does it engage a precise variety of resources. Instead, nanotechnology encompasses all facets of the fabrication of devices and systems by influencing material at the nanoscale. In education and the sciences, advances in nanotechnology and technology as a whole are blurring the lines between disciplines ( Schank, Krajcik, & Yunker, 2007 ).
Nanotechnology is truly a multidisciplinary field that includes individuals from chemistry, physics, biology, materials science, and engineering all working in a collaborative manner to better understand and apply knowledge of objects that meet the scale classification ( Clark & Ernst, 2005 ). Advancements in bioengineering, instrumentation, materials science, and manufacturing diminish the distinguishing characteristics of science and technological disciplines ( Jacobs, 1996 ). Rapid discovery, development, and advancement have resulted in relationships among science, technology, and society becoming increasingly stronger. Unfortunately, schools find it difficult to modernize curricula given the pace of innovation ( Fourez, 1997 ).
Progress in science and engineering at the nanoscale are critical for national security, prosperity of the economy, and enhancement of life quality for society as a whole. The National Science Foundation has identified that a major outcome goal of the United States is the development of “a diverse, competitive, and globally engaged U.S. workforce of scientists, engineers, technologists and well-prepared citizens ( 2004 , p. II-7).” It has been projected that approximately two million workers will be required worldwide during the next decade. Many science, engineering, and technology disciplines converge at the nanoscale, because they consist of many common principles and tools of investigation ( Rocco, 2002 ). By 2015, it is predicted that nano-related goods and services will be a market in excess of one trillion dollars ( Ratner & Ratner, 2003 ). Thus, nano businesses will become the fastest growing industry in history, outranking the telecommunications and information technology industries combined.
Nanotechnology will have an impact on war, crime, terrorism, law enforcement, and commercial goods ( Wilson, Kannangara, Smith, Simmons, & Raguse, 2002 ). The military has a broad interest in nanotechnology, which spans optical systems, nanorobotics, nanomachines, smart weapons, nanoelectronics, virtual reality, armors, energy-absorbing materials, and bionano devices. Quite possibly the most important areas of research and development concerning nanotechnologies are nano-optics and nano photonics. Advancement in these areas has potential to create new industrial and manufacturing processes and uncover new ways to maintain a clean and sustainable environment. Although much of the benefit of nanotechnology is anticipated, applications of nanotechnology are not all categorized as futuristic. Manufacturers are currently producing products with nanoproperties. Many materials in current use are products of nano-related research, such as stain repellents, water repellents, and dental fillers. Much of the invention and innovation in nanotechnology is dependent on associated processes. In order to manufacture at a smaller and smaller level, instruments and chemicals must be used with extreme precision. Industrial and manufacturing process applications of nanotechnologies represent the foundation of further discovery. These applications that merge biology, chemistry, medicine, and fabrication are broad-based areas that exhibit great potential for cross-disciplinary approaches to education.
Heightened need for targeted knowledge and skill will most certainly have an imprint on the educational system at all levels. The identified “blurring” of disciplines plays much into the emerging structure of an integrated systems approach in technology education classrooms across the country. Shields and Rodgers (2005) identified the need for heightened awareness of experimental technologies for technology education students. The competencies identified in their study are students’ abilities to recognize the ramifications of green energy, efficient vehicles, biometrics, and nanotechnology, many of which demonstrate a direct relationship.
A key challenge for nanotechnology development is the education and training of a new generation of skilled workers in the multidisciplinary perspectives necessary for rapid progress of the new technology. The concepts at the nanoscale (atomic, molecular and supermolecular levels) should penetrate the education system in the next decade in a manner similar to the way the microscopic approach made inroads in the last 50 years. Furthermore, interdisciplinary connections reflecting unity in nature need to be promoted. Such education and training must be introduced at all levels, from kindergarten to continuing education, from scientists to nontechnical audiences that may decide the use of technology and its funding. ( Roco, 2002 , pp. 1247-1248)
Technological discovery and improvement continues to unlock new content to incorporate into the classroom. Elevated subject matter and classroom experiences that feature cutting-edge approaches are essential to develop knowledge and motivate students at the K-12 level ( Sweeney, 2006 ). Sweeney further identifies that future progression in nanoscale science and engineering will rely on the content and quality of education in the K-12 classroom. Schank, Wise, Stanford, and Rosenquist (2009) concluded from a recent study that suitable preparation and guidance for teachers, paired with well-designed and engaging nanoscience curricular activities are necessary to facilitate student concepts of the fundamental principles that preside over the performance of particles on the nanoscopic scale.
Curricular activities that incorporate realworld examples can enhance students’ attitudes about science and emerging ideas. Jones, Andre, Superfine, and Taylor found in a 2003 study that students can increase their understanding of what the nanoscale is through development of high-quality three-dimensional graphics and utilization of virtual reality software. In a 2006 National Science Foundation project, Chizmeshya, Drucker, Sharma, and Carpenter identified that direct and virtual microscopy practice by students establishes scale knowledge at the nano level and provides a constructive source for computer modeling experiments. This grouping provides an opening for students to take part directly in nanoscale materials research at an appropriate age and with suitable content.
Scale remains one of the most difficult concepts for students to grasp regarding nanotechnology. Relating nanometers to customary units of measure provides a minimal level of awareness of actual nanoscale objects. However, the relation of visible and tangible objects that students perceive as extremely small can accentuate this knowledge for students. Table 1 is an example that standardized units as well as tangible, visible, or represented objects as comparisons to the nanoscale.
Note: Table information courtesy of the VisTE Project |
One meter = One billion (1,000,000,000) nanometers |
One inch = 25,400,000 nanometers |
A human hair = approximately 50,000 nanometers in diameter |
Bacteria (single-celled microorganisms) = from a few hundred to 1,000 nanometers across |
10 hydrogen atoms in a line = 1 nanometer |
Approaching scale through exponential means with a graphical representational basis is also an effective mode for approaching this type of material ( Wiebe, Clark, Ferzli, & McBroom, 2003 ). Clark and Ernst (2005) report findings of a three-year study linking science and technology concepts through the creation of visualizations. The study involved the creating, piloting, and field testing 12 instructional units for technology education in grades 6 through 12 (typically ages 11-18). The intent of forming instructional units in the US educational system is to organize information on a topic into lessons, taking into consideration time, materials, and preparations. The instructional units cover topics on agricultural and related biotechnologies, medical technologies, nanotechnologies, and principles of visualization related to fields studied in pre-engineering curricula. Study data indicates that students who participated in the instructional units involving the creation of visualizations significantly increased their knowledge in the identified content areas.
Implications for Teacher Preparation
Universities are beginning to include nanotechnology (i.e., content and practice) in their technology education teacher-preparation curricula. One specific program that includes a course partially dedicated to nanotechnology and its application is the Technology, Engineering, and Design Education Program at North Carolina State University in Raleigh: It is titled “Emerging Issues in Technology.” In this course, students explore contemporary medical, environmental, and biotechnological topics. They complete associated learning activities, experimentation/data collection exercises, and modeling projects. The medical technology sequence consists of disease prevention and medical imaging technologies, investigating pasteurization, irradiation, sterilization, water treatment, sanitation, immunization, computerized axial tomography, ultrasound technology, magnetic resonance imaging, and endoscope technology.
The environmental sequence is composed of graphical weather patterns, Earth observation systems, green power, sustainability, cradle to cradle design, and renewable energy resources. The biotechnology sequence consists of DNA technology, gene detection, enzyme replacement, cell culture, and associated nanotechnologies. A major portion of the biotechnology sequence is dedicated to the study of nanotechnology. Table 2 highlights the targeted areas of study within nanotechnology, the proportional emphasis dedicated to the specific unit, and the content of each area.
Nano Topics | Proportion | Content |
---|---|---|
Microscale and Nanoscale | 15% | “Nano” and nanometer, English expression, magnitude as a number and exponent, relative size |
Carbon Forms | 8% | Physical properties, oxidation state, compounds, carbon form uses |
Modeling and Simulation | 15% | Data structuring, process structuring with choice of 3DS applications, Flash applications, Truespace applications |
Water Purification | 8% | Contaminants, microfiltration, porous nanoparticles, nanotubes. |
Nanorobotics | 6% | Biosensors, control design, controlled manipulation, bio-uses |
Biomedical Applications | 12% | Drug delivery, bio-machines, biosensors |
Future Application | 12% | Fuel cells, solar cells, nanofibre armour |
Risks and Benefits | 12% | Security, health, environment |
Nano Ethics | 12% | Public safety, regulation, standards |
The nanotechnology unit represents approximately 30 percent of the Emerging Issues in Technology course. Additionally, the Technology Engineering and Design Education Program offers courses in Mechatronics, Scientific Visualization, and Technical Animation. The Mechatronics course expands on nanorobotics Animation courses greatly contribute to the nanotechnology modeling and simulation requirements in the Emerging Issues in Technology course. An animation still of an atomic force microscope used to manipulate atoms is shown in Figure 1. Nanomanipulation, or the ability to move atoms individually and arrive at predetermined arrangements, is an important first step in realizing the potential of nanotechnology.
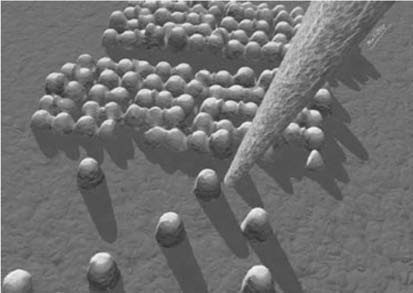
Nanomanipulation is just one aspect of nanotechnology that can be enhanced with application when interwoven throughout coursework in teacher-preparation programs.
A key to implementing the study of nanotechnology content and processes into a technology education teacher-preparation program is to provide an integrated and spiraling sequence of course offerings. These offerings should gradually build upon one another and establish layered content knowledge and performance-based application that merges identified systems-based benchmarks in a logical and connected flow. Additionally, visual examples and simulated real-world applications should be used where possible to enhance students’ engagement and understanding in technology teacher education. The use of intrinsically motivating approaches, such as visual and kinesthetic learning methods, creativity strategies, problem-based learning, and learning through design are particularly effective methods for reinforcing STEM-based material ( Clark & Ernst, 2007 ). Problem-based and project-based approaches to student learning also have been shown to improve the understanding of basic concepts and to encourage deep and creative learning despite academic content area ( Powers & DeWaters, 2004 ). The identification of ideas derived from mathematics and science enables instructional sequences to visibly reinforce applications of concepts, skills, and principles through identified content. In this case, chemistry, physics, biology, materials science, and engineering are all disciplines central to the study, experimentation, and further development of nanotechnologies.
Conclusion
Steadfast development of new technologies leading to continual transformation of society serves as a strong indicator that current educational practices should be altered in order to prepare knowledgeable and engaged citizens. Contemporary approaches and practices to further engage learners and enhance their abilities to apply nanoscale-related content knowledge at the highest level must be developed continually, in order for the United States to solidify itself as the primary mover of nanotechnology research and development.
Modeling and visualizations reinforce associated scientific and technological concepts. The use of these associated communication technologies heightens student involvement and strengthens individual technological and scientific knowledge and abilities while providing students with an opportunity to gain a firm grasp of engineering principles behind the technologies ( Newhagen, 1996 ). Implementing enhanced instructional tools and methods in technology and science classrooms in a coordinated structure has the potential to build content understanding of not only nanotechnology, but many of the “blurred” areas identified by Schank, Krajcik, and Yunker in their 2007 work. A true multidisciplinary approach that incorporates computer graphics and applications has been demonstrated to be effective in classroom instruction associated with nanoscience and nanotechnology ( Chizmeshya, Drucker, Sharma, & Carpenter, 2006 ; Jones, Andre, Superfine, & Taylor, 2003 ; Clark & Ernst, 2006), but this approach has much more to offer technology/science and education as a whole if its proponents are provided adequate tools and support on a larger scale.
Dr. Jeremy V. Ernst is an assistant professor in the Department of Math, Science, and Technology Education at North Carolina State University, Raleigh. He is a member of the Gamma Tau chapter of Epsilon Pi Tau.
References
Chizmeshya, A. V. G., Drucker, J., Sharma, R., & Carpenter, R.W. (2006). Real time nanostructure imaging for teaching nanoscience, and nanotechnology. Boston, MA: Material Research Society (0931-KK03-07).
Clark, A. C. & Ernst, J. V. (2005). Supporting technological literacy through the integration of engineering, mathematic, scientific, and technological concepts. Published Proceedings of the American Society for Engineering Education Annual Conference and Exposition, Session 146. Chicago, IL.
Clark, A. C. & Ernst, J. V. (2007). A model for the integration of science, technology, engineering, and mathematics. The Technology Teacher, 66 (4), 24-26.
Fourez, G. (1997). Scientific and technological literacy as a social practice. Social Studies of Science, 27, 903-936.
Jacobs, J. A. (1996). Designing the very small: Micro and nanotechnology. The Technology Teacher, 55 (8), 22-27.
Jones, M. G., Andre, T., Superfine, R., & Taylor, R. (2003). Learning at the nanoscale: The impact of students’ use of remote microscopy on concepts of viruses, scale, and microscopy. Journal of Research in Science Teaching, 40 (3), 303-322.
Lakhtakia, A. (2006). Priming pre-university education for nanotechnology. Current Science, 90 (1), 37-40.
Mnyusiwalla, A., Daar, A. S., & Singer, P. A. (2003). “Mind the gap”: science and ethics in nanotechnology. Nanotehcnology. 14 , R9-R13.
National Science Foundation. (2004). National science foundation fy 2004 performance and accountability report. Arlington, VA: Author.
Newhagen, J. E. (1996). Why communication researchers should study the Internet: A dialogue. Journal of Communication, 46 (1), 4-13.
Powers, S. E. & DeWaters, J. (2004). Creating project-based learning experiences university-k-12 partnerships. Published Proceedings of the American Society for Engineering Education Frontiers in Education Conference, Session F3D. Savannah, GA.
Ratner, M. & Ratner, D. (2003). Nanotechnology: A gentle introduction to the next big idea. Upper Saddle River, NJ: Prentice Hall.
Roco, M.C. (2002). Nanoscale science and engineering education activities in the United States. Journal of Nanoparticle Research, 4 , 271-274.
Schank, P., Krajcik, J., & Yunker, M. (2007). Can nanoscience be a catalyst for educational reform?. In F. Allhoff, P. Lin, & J. Moor (Eds.), Nanoethics: The ethical and social implications of nanotechnology. (pp. 277-290). Wiley.
Schank, P., Wise, P., Stanford, T., & Rosenquist, A. (2009). Can high school students learn nanosciene? An evlaluation of the viability and impact of the nanosense curriculum. Menlo Park, CA: SRI International.
Shelley, T. (2006). Nanotechnology: New promises, new dangers. New York: Zed Books.
Shields, C. J. & Rodgers, G. E. (2005). Incorporating experimental technologies in the middle level technology education classroom. Journal of Industrial Teacher Education, 42 (4), 72-80.
Sweeney, A.E. (2006). Teaching and learning in nanoscale science and engineering: A focus on social and ethical issues and k-16 science education. Material Research Society (0931-KK03-05). Boston, MA.
The National Academies. (2006). A matter of size (National Academy of Science Publication) Washington, DC: The National Academies Press.
Wiebe, E. N., Clark, A. C., Ferzli, M., and McBroom, R. (2003). The VisTE Project: Visualization for improved technological and scientific literacy. Published Proceedings of the American Society for Engineering Education Annual Conference and Exposition, Session 2438. Nashville, TN.
Wilson, M., Kannangara, K, Smith, G., Simmons, M., & Raguse, B. (2002). Nanotechnology: Basic science and emerging technologies. Washington, DC: Chapman and Hall/CRC.
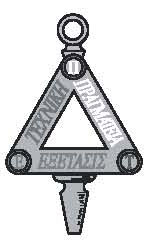
TS