Staking the Claim for the 'T' in STEM
Todd Kelley
Introduction
There are a number of examples in technology education history of multidisciplinary and interdisciplinary efforts linking technology education with other disciplines; however, there has never been a time in technology education where multidisciplinary and interdisciplinary efforts are not only promising but also may be essential for the prosperity of technology education. One important example of blurred boundaries caused by a multidisciplinary effort from our recent past was the Math, Science, and Technology (MST) movement in the early 1990s. The MST movement had an important impact on technology education, and a strong case can be made that the MST efforts of the 1990s paved the way for the recent STEM education initiatives. However, in this article, the author will seek to make the case that no previous multidisciplinary and interdisciplinary efforts within technology education's history has such potential to impact the field greater than the recent Science, Technology, Engineering, and Mathematics (STEM) movement. Here, the terms multidisciplinary and interdisciplinary will be defined, a recent history of such efforts in technology education will be reviewed, how funding can and has blurred the mission of technology education will be explored, and the opportunities for technology education regarding STEM education will be presented.
Multidisciplinary and Interdisciplinary defined:
The terms multidisciplinary and interdisciplinary have become educational "buzzwords," and these terms have been misused, abused, and often used interchangeably, thus, adding to the confusion of how these terms are used in practice.
Multidisciplinary has been defined as "individuals from different disciplines working independently on different aspects of a project" ( Mallon & Burton, 2005 , p. 2).
Interdisciplinary understanding has been defined as "the capacity to integrate knowledge and modes of thinking drawn from two or more disciplines to produce a cognitive advancement-for example, explaining a phenomenon, solving a problem, creating a product, or raising a new question-in ways that would have been unlikely through single disciplinary means" ( Mansilla, 2005 , p.16).
The MST Movement
The United States Industrialist Henry Kaiser once said, "Trouble is only opportunity in work clothes" ( Phillips, 1993 , p. 8). The MST movement began as a result of trouble within U.S. schools, specifically the need to improve American students' scores in math and science. National reports such as Everybody Counts: A Report to the Nation on the Future of Mathematics Education ( National Research Council, 1989 ) and Project 2061: Science for All Americans ( American Association for Advancement of Science, 1989 ) documented the need to improve students' knowledge and understanding of math and science. Likely some members within technology education believed the MST movement was an excellent opportunity for technology education to position itself as a necessary discipline for all learners and provide a necessary funding source for technology education. Householder (1992) indicated that technology teacher education had an excellent opportunity in the MST movement to locate funding for undergraduate education that was once only possible for science and mathematics education; whereas other technology education leaders indicated that MST would elevate the status of the field ( LaPorte & Sanders, 1993 ). Equally, technology education leaders questioned technology education's chances of survival with two core disciplines ( Foster, 1994 ; Gloeckner, 1991 ). Gloeckner (1991) provided examples of possible roadblocks that would limit the success of the MST movement for technology education. Moreover, Daugherty and Wicklein (1993) conducted research to determine the perceptions held by math, science, and technology teachers' of technology education. The results revealed that they were poor and that misconceptions abound regarding technology education.
One project that emerged out of the MST movement was the New York State Technology Education Network (NYSTEN), which was funded by the NSF to improve the quality of technology education in that State. The NYSTEN project was designed to provide contemporary technological, pedagogical, and leadership enhancement to technology education teachers across New York state ( Burghardt & Hacker, 2002 ). This author was a member of the NYSTEN project as a secondary technology teacher during the 1994-1996 school years. Through the experiences of piloting a MST middle school project and assisting in the writing of a middle school MST project assessment instrument, author witnessed the removal of barriers among math, science, and technology education teachers. Furthermore, a new social network of educators was formed as in-service teachers from math, science, and technology education disciplines gathered for summer professional development workshops and to draft MST curriculum and MST standards for New York State ( Liao, 1998 ). However, once federal funds ran out, such as Goals 2000 monies, the MST movement lost critical momentum and the MST standards project for New York State has never been fully implemented as designed. Certainly, other factors were at work regarding the New York State MST movement beyond a funding issue. The New York State Department website states:
Through the foresight of many, the standard for technology and technology education programs was linked to mathematics and science. Illustrating the interconnectedness of these three subjects the Mathematics, Science, Technology (MST) Learning Standards has created a dynamic force for demonstrating student knowledge. While mathematics and science have had a long history in education, technology education is a relatively new subject with less stature and acceptance. Added to this the testing pressures placed on mathematics and science education, technology education has been overlooked as a tool for improving student achievement ( NYS Technology Education Framework Initiative, 2006 , p. 4).
This statement supports the positions of Gloeckner (1991), Foster (1994) , and others who warned of the dangers of technology education becoming the stepchild in a bad marriage of math and science. Technology educators must learn from these past events in order to provide a vital case for technology education in the STEM movement.
The Blurs of Funding
There are countless examples of educational funding providing a "blurring of the boundaries" effect within the field of technology education. In fact, researchers can go all the way back to the days of Woodward and the manual training movement to see a prime example of "border crossing." Often, those who view technology education as a part of general education are sometimes forced to consider a compromise when faced with the idea of missing out on federal funding that supports career and technical education. Lewis (1996) uses Woodward as an example of one who was forced to compromise his ideals for manual arts for all children's general education. Woodward possessed a liberal education viewpoint of manual training that moved way beyond manual training as trade training; however, with the passing of the Smith-Hughes Act of 1917, Woodward was faced with a "border crossing." Supporters of manual training viewed it as a way to loosen a union stranglehold on apprenticeship; consequently, Woodward and his "camp" were forced to advertise manual training as vocational training and not a liberal education for all in order try to gain Smith-Hughes monies. This "fence sitting approach" has been a sore spot in the field of technology education for some time ( Karnes, 1999 ). Although this "blurring of borders" is related to the mission or purpose of technology education, it does serve as an example of the dangers of following the funding and the affect that it has on a discipline. This could be a root cause for why some educators have indicated that technology education has failed to establish its mission ( Wicklein, 2006 ).
It is with careful consideration of our past regarding "border crossing" that technology education leaders should count the cost of seeking federal STEM dollars. Currently, 85 NSF programs include STEM somewhere in the RFP solicitation (NSF Website, date accessed 8/29/09). The current $787 billion stimulus package contained $100 million for the National Science Foundation ( Riley, 2009 ). Sanders (2009) pointed out that during the past 20 years there has been major education reform through major professional associations and crediting boards regarding science, mathematics, technology, and engineering disciplines (e.g., AAAS, 1989 , 1993 ; Peterson, 1996 ; ITEA, 1996 , 2000 ; NCTM 1989 , 2000 ; NRC, 1994 ), illustrating the massive efforts to improve STEM education. Unquestionably, a great opportunity exists for those in technology education to conduct research in STEM education. However, there are other opportunities for technology educators to capitalize on STEM initiatives beyond simply research efforts. The National Science Board identified the critical need for STEM teachers within the next decade. "In the next decade, the Nation is going to need "2.2 million new teachers in K-12 schools and community education settings. The greatest need now and into the future is for teachers in the STEM areas" ( National Science Board, 2007 , p. 1). Undoubtedly, technology teacher educators have an excellent opportunity to focus on the preparation of pre-service STEM teachers. It may be appropriate for technology educators to consider revamping technology teacher education programs to ensure that technology teacher education takes ownership of the T in STEM.
Blurring of the T in STEM
Certainly, a blurring of the boundaries within the STEM acronym includes an interpretation of the T in STEM. How is technology defined by those proposing a STEM approach to education? Most if not all technology educators would suggest that technology education is the T in STEM. However, outside our field do other educators, educational policymakers, school officials, and the holders of educational funding recognize that we deliver the T in STEM? Does the general public know what the T in STEM is? It is well documented that technology educators have struggled to define the discipline ( Wicklein, 2006 )? Technology educators have struggled with the field's purpose or mission, which may have been a result of "border crossing" ( Lewis, 1996 ). There has even been a struggle with the name "technology education" ( Clark, 1989 ). These factors have caused some to suggest that technology education struggles with its identity. To illustrate this point, when someone asks what a technology educator does for a living, much of that conversation is about what the person is "not." They also note that technology education should not be confused with educational technology and that many technology educators are former Industrial Arts or shop teachers, but they are not that anymore. It is no secret that technology educators, struggle to define who they are. Furthermore, it is important to recognize that they currently do not own the T in STEM from the general public's perception. Education Week (March, 27th, 2008) published an entire issue centered on STEM education where throughout the publication were success stories of STEM education around the nation. If one reads the articles in depth, most of the examples of the T in STEM education are representative of informational technology or educational technology more than they reflect technology education practice. Moreover, when the national scorecard reports on STEM are examined, the T (technology) is measured by counting the number of computers schools had accessible for student use. One article in the Education Week STEM issue was titled, "Where Is the T in STEM?" ( Cavanagh, 2008 ). In this article, experts debated if the practical application of math and science was getting the national attention it deserved. These examples continue to cause members of technology education to wonder if the field will ever be recognized as the T in STEM and it furthers the misconceptions of technology education and fosters dissention among STEM disciplines. Now is the time to raise the all-important question: How can technology educators stake their claim on the T in STEM?
Staking the Claim for the T in STEM
A key for defining the T in STEM is research . In order for the field of technology education to be known as the T in STEM separate from the science, math, and engineering community, it must rely on quality, relevant research. Quality research results speak to educational leaders and policymakers in a way that is meaningful and powerful. Technology education has countless publications that are little more than PR pieces of success stories related to effective outcomes indicating that students become motivated to learn because of technology education classes. Although there are many examples of the power of project- and design-based instruction, this does not mean much to educators outside the field of technology education until research confirms it. It is not enough to say that students are engaged and excited about learning because of technology education programs. It is not enough to say that kids are finally connecting their science and math skills because of technology education. Technology education doesn't need more public relations pieces regarding its value; it needs more research studies to get at the core of how technology education improves learning. It is well documented that this field lacks quality rigorous research. From Sanders in 1987 to more recent reports from Foster (1992) , Petrina (1989), Zuga, (2000) and Lewis (1999) , all documented a great need for more research in the field of technology education. Interestingly, Gloeckner (1991) accurately indicated that one of the major roadblocks at that time for technology education's success with the MST movement was the lack of funding for educational research. Today, there has never been a better time to conduct research on interdisciplinary education efforts because of the STEM movement. This movement is not simply another example of the latest pedagogical strategy to improve American students' scores in math and science; some experts suggest that STEM education has major national, economic, and security implications. Chairman of the National Science Board sent a one-page letter on 11 January 2009, to President-Elect Obama as a call to action regarding the improvement of STEM education for all students. In this letter, Chairman Beering stated:
Our national economic prosperity and security requires that we remain a world leader in science and technology. Pre-college STEM education is the foundation of that leadership and must be one of our highest priorities as a Nation. We urge the new Administration to seize the opportunity provided by this special moment in history and mobilize the Nation to support the development of high quality STEM knowledge and skills for all American students. It is essential that we act now to ensure all of our children and American society as a whole can continue to prosper in the 21st century technology-based economy (National Science Board, 2009).
The letter also identified core components of an effective STEM educational system, including promoting student learning in STEM that encourages critical- thinking, communication, and problem solving-skills, increases to teacher salaries to recruit and retain high-quality STEM teachers, provides advanced technologies for the classroom, and provides federal funding for peer-reviewed and competitively funded research on the learning and teaching of STEM education.
K-12 Design-based Instruction Research (Outside Technology Education)
Technology educators should learn from researchers outside of the field whom are researching education constructs that align with technology education's traditional pedagogical approaches, including project-based instruction, design-based instruction, and service-learning approaches to design and problem solving. For example, recently project-based learning has been successfully implemented in science classrooms to improve science instruction and develop skills of scientific inquiry ( Krajcik & Blumenfeld, 2006 ). Although project-based learning (PBL) is not exclusively a design-based approach, the learning theories align, and many project-based learning experiences contain an embedded design approach. Research confirms that project-based learning has been successful at increasing students' tests scores compared to student test scores in traditional classrooms ( Marxet al., 2004 ). Furthermore, research on project-based science instruction revealed that this instructional approach could help all students regardless of culture, race, or gender ( Atwater, 1994 ; Geier, et al., 2008 ; Haberman, 1991 ).
Researchers at Tuffs University and Boston College (Rogers, Jarvis, & Barnett, n.d.) have developed engineering-based science units for grades 3 and 4 using LEGO™ kits. The projects' focus was to infuse engineering concepts into elementary education while improving the teaching and learning of science. Case-based reasoning is another approach to design-based instruction. Kolodner (2006) developed an approach to design-based instruction based on a case-based reasoning theory. Kolodner named this middle school level educational approach to project-based inquiry Learning by Design . The Learning by Design approach engages students into two essential cycles: design/redesign and investigate/explore. Students experience a variety of doing and reflecting activities and share their new knowledge in public presentations as a way to help students process these experiences and make connections with core content knowledge ( Kolodner, 2006 ). Technology educators would be best served by learning from educational researchers outside technology education whose examining constructs align well with their own pedagogical approaches. Some examples of educational research have come from technology educators who have found ways to acquire funding that aligns with STEM initiatives. For instance, Burghardt and Hacker (2003) acquired funding from an NSF's Math Science Partnership (MSP) grant to develop a contemporary pedagogical approach to design-based instruction developed for middle school technology education. Hacker and Burghardt's informed design approach allows the designer to move beyond trial-and-error problem solving that often leaves students with a lack of understanding about why the design solutions succeeded or failed. Burghardt and Hacker (2004) indicated that the informed design approach emphasizes the use of design challenges that require the application of math and science concepts through an engineering design approach in order to develop design solutions. Burghardt and Hacker's project serves as an example of an interdisciplinary (STEM) approach to engineering design-based instruction that illustrates the benefit of the "blurring of the interdisciplinary boundaries."
Making the Connection: Engineering Design and Science Inquiry
These recent science educational research findings have indicated effectiveness of using an engineering design-based approach to enhance the teaching of science inquiry ( Kolodner, 2006 , Krajcik & Blumenfeld, 2006 ). One problem remains regarding locating a logical theoretical approach to STEM education that will leverage technology education's long history with design-based instruction while at the same time retaining the core purpose: promoting technological literacy. Sanders (2009) proposed a pedagogy called "Purposeful design and inquiry" that combines technological design with science inquiry situated in the context of technological problem solving. It is clear that Sanders identifies the connection between science inquiry and design; however, the current technological design process models do not specifically engage in science inquiry or mathematical analysis ( Hailey, Erekson, Becker, & Thompson, 2005 ). Sanders also indicated that technology education teachers like to boast about teaching science and mathematics but often fail to do so in practice. This is quite possible because they are using a design process that does not demand engagement with math and science; thus, design practice in technology education reverts to a trial-and-error method. Lewis (2006) identified the conceptual parallels of design and inquiry which provides an ideal "border crossing" for technology education and science education. Lewis identified the convergences and divergences of design and inquiry, and although much of Lewis's examples of design are centered around the engineering design process and engineering design practice ( Koen, 1985 ; Petroski, 1994 ) Lewis never uses the term engineering design when he writes about design in this article. This author proposes that the infusion of engineering design as a logical approach to STEM education can allow technology educators to remain true to core principles and advance the STEM education cause. Engineering design provides students with a systematic approach to solving problems that not only can be used in science and engineering applications but also in many other interdisciplinary learning experiences. A graphic created with a simple combination of gears provides an illustration of this concept (see Figure 1). Using situated learning as the driver (driving gear) to engage in the engineering design process and science inquiry simultaneously is one approach to illustrate the natural engagement between the engineering design process and science inquiry. Technology education can build upon recent research results of project-based instruction, case-based instruction ( Kolodner, 2006 ; Krajcik & Blumenfeld, 2006 ) and other engineering design pedagogical approaches ( Burghardt & Hacker, 2004 ) to blend the best of these approaches to the context of engineering design. Project-based instruction research reveals that students' motivation for learning increases when allowing students to build physical artifacts, a pedagogical approach that technology educators have used successfully for years. Infusing an engineering design approach to science instruction through inquiry not only will enhance the students' ability to apply science knowledge and scientific discoveries but will also help them to apply their mathematics knowledge to inform the design process. The analytical element of the design process allows students to use mathematics and science inquiry to create and conduct experiments that will inform the designers about the function and performance of potential design solutions before a final prototype is constructed. This approach to engineering design learning will consist of authentic design tasks that allow students to build upon their own experiences and provide opportunities to construct their own new science and math knowledge through design analysis and scientific investigation. Consider situation learning as a driving gear that engages science inquiry and the engineering design process simultaneously through an authentic engineering design challenge. An authentic approach to engineering design will use science inquiry and mathematical analysis to inform the designer as he or she works through the engineering design process.
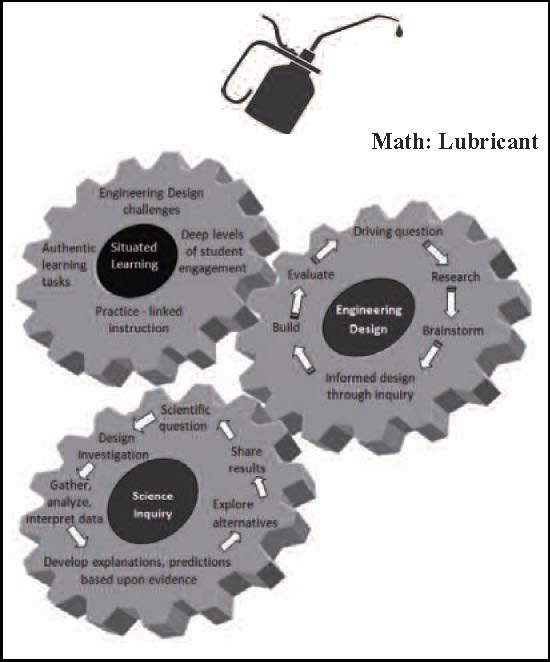
Figure 1. Relationship between Situated Learning, Engineering Design, and Science Inquiry. Assumptions: engineering design process inspired by Eide et al. (2001) revised to provide elementary age appropriate terms. The iterative process of engineering design is assumed but is not captured in this graphic.
Science inquiry may be a new term to some in technology education; however Lewis (2006) provides detailed description of science inquiry and the conceptual parallels to design creating a natural link between K-12 science and technology education. One example of a curriculum project that links science inquiry and design within technology education is an NSF-funded and ITEA-implemented curriculum project for the elementary grades (grade 5-6) is the I3 Invention-Innovation-Inquiry project. The I3 curriculum is designed to promote technological literacy through 10 units, which are standards-based learning activities engaging students in brainstorming, visualizing, testing, refining, and assessing technological designs. One unit specifically used science inquiry as a part of the design and problem-solving process (International Technology Education Association, n.d.). Clearly these curriculum developers are acknowledging the interrelationship between science inquiry and the engineering design process. Examples such as this one illustrate an approach to subject integration that captures the true essence of STEM education.
Promising Ventures in STEM education
Program revisions . There are many opportunities for technology teacher education programs to engage in STEM education. Some institutions have already altered their technology education teacher degree programs to address multidisciplinary and interdisciplinary needs, and others have approached these needs by providing opportunities for interdisciplinary endorsements for nonmajors (Virginia Tech, College of New Jersey). It is extremely important that technology teacher educators remain progressive in their approach to prepare pre-service teachers for the current conditions of secondary technology education. With more and more emphasis on STEM initiatives and movements to include engineering design as a logical vehicle for technology education to deliver STEM learning experiences, it is an appropriate time for accreditation boards such as NCATE to revisit their standards. These have been heavily influenced by a curriculum theory and conceptual framework that is nearly 30 years old ( Snyder & Hales, 1981 ). Numerous research studies involving in-service and preservice professional development opportunities can be used to prepare future K-12 STEM teachers; simultaneously research could be conducted to determine the appropriate levels of content and pedagogical content knowledge necessary to effectively team STEM ( Brophy, Klein, Portsmore, & Rogers, 2008 ). Results from these studies along with research studies specific to the technology education field can provide vital information necessary to reform programs.
Informal education partnerships . One interdisciplinary venture that is very promising for technology that aligns naturally with STEM education is collaboration with Science/Technology museums. A number of educational research efforts have linked Science/Technology museums with university research efforts. This collaborative effort is a potential win-win for both institutions. One excellent museum/university approach is to field test design-based curriculum projects. Furthermore, there are many opportunities for informal educational research conducted at museums that reduces Internal Review Board (IRB) restrictions often encountered when a university is partnered with a K-12 school district. One example of informal education research partnership is the Engineering Our Future New Jersey a pilot project that partnered Stevens Institute of Technology with the Boston Museum of Science. Stevens Institute of Technology provided professional development opportunities for a dozen elementary teachers to help them prepare to implement the Boston Museum of Science's Engineering is Elementary (EiE) curriculum. The project sought to identify the impact of a pre-engineering curriculum on a student's understanding of technology and engineering concepts. Shields (2006) research on EiE curriculum materials has revealed strong gains in pre-post test scores and assessment within math and science have occurred in Colorado, Florida, Minnesota, Massachusetts, and New Jersey.
Community college, regional institutions, and research-focused partnerships.
The NSF's Advanced Technological Education (ATE) solicitation seeks research proposals with an emphasis on two-year colleges with a focus on education of technicians for high-technology fields that drive our nation's economy (National Science Foundation, n.d.). Opportunities like this RFP illustrate the shift in paradigm regarding research-focused universities. It appears that the NSF along with much of the post-secondary education community at large are moving away from a mindset that community colleges, regional institutions, and teachers' colleges are focused only on teaching to a new frame of mind that embraces research at all levels. One university with a long history as a teachers' college recently hired a president who quickly established a strategic plan to establish a strong research agenda to acquire external funding. The days of leaving research for only the land grant R-1 research institutions is a thing of the past. Research funding opportunities, such as the NSF's ATE program, provide an opportunity for colleges and universities at all levels to establish an interdisciplinary approach to STEM education in a way that leverages each institution's strengths.
No child left behind: School improvement plans through interdisciplinary efforts . In-service technology teachers have an excellent opportunity through the annual "school improvement plan" process required by NCLB legislation to improve students' standardized test scores, and regardless of how the NCLB legislation continues to be reformed and renamed, school accountability through government legislation is here to stay. All teachers are required to join a school improvement team charged to create documentation of how schools plan to improve learning in standardized-tested disciplines. School improvement teams provide technology education teachers opportunities to establish partnerships with science and mathematics teachers in order to establish plans to infuse science and mathematics concepts into existing technology education curriculum. Technology education provides a logical context for teaching math and science concepts, and often these concepts are already embedded in the technology curriculum or design activities. The school improvement report provides opportunity to document these subject integration efforts. Furthermore, establishing a partnership with math, science, and technology education teachers on these school improvement teams also provides the technology teacher with the opportunity to create powerful allies. The partnerships established through school improvement plans can generate healthy dialogues that in turn can shatter misconceptions and create positive perceptions about technology education ( Daugherty & Wicklein, 1993 ).
Conclusion
The goal of this article was to present a strong case that no previous multidisciplinary and interdisciplinary efforts in technology education's history has such potential to impact the field greater than the recent Science, Technology, Engineering, and Mathematics (STEM) movement. A review of literature within technology education during the MST movement revealed a variety of perspectives regarding multidisciplinary and interdisciplinary efforts for technology education. Furthermore, opponents of MST for technology education identified potential pitfalls and dangers of partnering with math and science disciplines, and much of this can be revisited as possible concerns to consider within the current STEM movement. A case was made for technology education to stake the claim for the T in STEM by building a strong research agenda focused on STEM issues. Finally, promising ventures for technology education within STEM education were identified, including research funding sources, such as the NSF's Advanced Technological Education (ATE) to partner with two-year technical colleges, partnerships with local science and technology museums, and partnerships within schools' NCLB school improvement interdisciplinary groups. The final question unanswered remains: Who should lead these interdisciplinary efforts within technology education? The author purposefully featured a variety of STEM opportunities for technology education that include all levels of the technology education community. In order to stake the claim in the T in STEM, all members of technology education need to engage in these interdisciplinary opportunities. No one knows if STEM will rejuvenate the field of technology education, but if the members of this field are interested in becoming key players in STEM education, technology education educators must stake their claim now!
Dr. Todd Kelley is an Assistant Professor in the College of Technology at Purdue University, Indiana. He is a member of Phi chapter of Epsilon Pi Tau.
References
ABET Engineering Accreditation Commission. (2004). ABET criteria for accrediting engineering programs . Baltimore, MD: Author.
American Association for Advancement of Science. [AAAS]. (1993). Benchmarks for science literacy, Project 2061 . Washington, DC: Author.
American Association for Advancement of Science. (1989). [AAAS]. Project 2061: Science for all Americans . Washington, DC: Author.
Atwater, M. (1994). Research on cultural diversity in the classroom. In D. L. Gabel (Ed.), Handbook of research on science teaching and learning (pp. 558-576). New York: Macmillan.
Brophy, S., Kelin, S., Portsmore, M., & Rogers, C. (2008). Advancing engineering education in P-12 classrooms. Journal of Engineering Education , 369-382.
Burghardt, D. & Hacker, M. (2002). Large-scale teacher enhancement projects focusing on technology education. Journal of Industrial Technology Education, 39 (3), 88-103.
Burghardt, M. D., & Hacker, M. (2004). Informed design: A contemporary approach to design pedagogy as the core process in technology. The Technology Teacher , 64, 6-8.
Burghardt, M. D., & Hacker, M. (2003). The New York State Curriculum for Advanced Technological Education . www.nyscate.net.
Cavanagh, S. (2008). Where is the 'T' in STEM? Education Week 27 (30), 17-19.
Clark, S. C. (1989). The industrial arts paradigm: Adjustment, replacement, or extinction? Journal of Technology Education, 1 (1), 1-9.
Daugherty, M., & Wicklein, R. (1993). Mathematics, science, and technology teachers' perceptions of technology education. Journal of Technology Education, 4 (2), 28-43.
Education Week, (2008, March 27). STEM, the push to improve Science, Technology, Engineering, and Mathematics, Education Week, 27 (30). From http://www.edweek.org/ew/toc/2008/03/27/index.html
Eide, A.R., Jenison, RD., Marshaw, L.H., & Northrup, L. (2001). Engineering fundamentals and problem solving (4th ed.) Boston: McGraw-Hill.
Foster, P. (1994). Must we MST? Journal of Technology Education, 6 (1), 76-84
Foster, W. T. (1992). Topics and methods of recent graduate student research in industrial education and related fields. Journal of Industrial Teacher Education, 30 (1), 59-72.
Geier, R., Blumenfeld, P., Marx, R., Krajcik, J., Fishman, B., & Soloway, E. (2008). Standardized test outcomes of urban students participating in standards and project based science curricula. Journal of Research in Science Teaching, 45 (8), 922-939.
Gloeckner, G. (1991). The integration of science, technology, and mathematics myth or dream? Journal of Technology Education, 2 (2).
International Technology Education Association. [ITEA]. (1996). Technology for all Americans: A rationale and structure for the study of technology . Reston, VA: Author.
International Technology Education Association. [ITEA]. (2000/2002) Standards for technological literacy: Content for the study of technology . Reston, VA: Author.
International Technology Education Association. [ITEA]. (n.d.). I 3 Project: Inquiry, innovation, inquiry units for technological literacy, grades 5-6 . from http://www.iteaconnect.org/i3/index.htm
Hailey, C. E., Erickson, T., Becker, K., & Thomas, T. (2005). National center for engineering and technology education. The Technology Teacher, 64 (5), 23-26.
Householder, D. (1992). Redesign of technology teacher education: Model programs for the future. In Camelback Symposium: Critical issues in technology education . Reston. VA: International Technology Education Association.
Haberman, M. (1991). The pedagogy of poverty versus good teaching. Phi Delta Kappan, 73 (4), 290-294.
Karnes, M. R. (1999). Technology education in prospect: perceptions, changes, and the survival of the profession. Journal of Technology Studies, 15 (1), 12-35.
Koen, B. V. (1985). Definition of the engineering method . Washington, DC: American Society for Engineering.
Kolodner, J. L. (2006). Case-based Reasoning. In K. L. Sawyer (Ed.), The Cambridge handbook of learning sciences (pp. 225-242). Cambridge: Cambridge University Press.
Krajcik, J. S., & Blumenfeld, P.C. (2006). Project-based learning. In K. L. Sawyer (Ed.), The Cambridge handbook of learning sciences (pp. 317-333). Cambridge: Cambridge University Press.
LaPorte, J., & Sanders, M. (1993) Integrating technology, science, and mathematics in the middle school. The Technology Teacher, 52 (6), 17-21.
Lewis, T. (1996). Accommodating border crossings. Journal of Industrial Teacher Education, 33 (2), 7-28.
Lewis, T. (1999). Research in technology education-Some areas of need. Journal of Technology Education, 10 (2), 41-56.
Lewis, T. (2006). Design and inquiry: Bases for an accommodation between science and technology education in the curriculum? Journal of Research in Science Teaching, 43 (3), 255-281.
Liao, T. (1998, Summer-Fall). Ideas, technology literacy: moving beyond mathematics, science, and technology (MST) integration. Journal of Technological Studies , 52-64.
Mallon, W. T., & Burnton, S. (2005, June). The functions of centers and institutes in academic biomedical research. Analysis in Brief 5 (1), 1-2.
Mansilla, V. B. (2005). Assessing student work at disciplinary crossroads. Change, 37 (1) 14-21.
McKay, M., Macalalag, A., Shields, C., Brockway, D., & McGrath, B. (2008). Partnership to improve student achievement through real world learning in engineering, science, mathematics and technology . American Society for Engineering Education.
Marx, R. W., Blumenfeld, P. C., Krajcik, J.S., Fishman, B., Soloway, E., Geier, R. & Revital, T.T. (2004). Inquiry-based science in the middle grades: Assessment of learning in urban systemic reform. Journal of Research in Science Teaching, 41 (10), 1063-1080.
National Council of Teachers of Mathematics. [NCTM]. (2000). Principles and standards for school mathematics . Reston, VA: Author.
National Council of Teachers of Mathematics. [NCTM]. (1989). Curriculum and evaluation standards for school mathematics . Reston, VA: Author.
National Research Council. [NCR]. (1994). National science education standards . Washington, DC: National Academy Press.
National Research Council [NRC]. (1989). Everybody counts: A report to the nation on the future of mathematics education . Washington, DC: National Academy Press.
National Science Foundation, Advanced Technological Education program. Retrieved on from http://www.nsf.gov/funding/pgm_summ.jsp?pims_id=5464 National Science Board, Minutes from the 396TH MEETING NATIONAL SCIENCE BOARD, March 2007. Retrieved from http://www.nsf.gov/nsb/meetings/2007/0208/minutes.pdf
New York State Education Department. (2006). NYS Technology Education Framework Initiative, pp 4. from http://www.emsc.nysed.gov/cte/technology/initiative/home.html
New York State Education Department. (1996). Learning standards for mathematics, science, and technology . Albany, NY: Author.
Peterson, G.D. (1996). Engineering criterion 2000: The ABET vision for change. JOM, 48 (9), 12-14.
Petrina, S. (1998). The politics of research in technology education: A critical content and discourse analysis of the Journal of Technology Education, Volumes 1-8. Journal of Technology Education, 10 (1), 27-57.
Petrina, S. (1993). Diversity, not uniformity; United, not standardized: A reaction to Wright's "Challenge to all technology educators." Journal of Technology Education, 4 (2), 71-79.
Petroski, H. (1994). Design paradigms: Case histories of error and judgment in engineering. Cambridge, England: Cambridge University Press.
Phillips, B. (1993). Phillip's book of great thoughts and funny sayings . Carol Stream, IL: Tyndale House.
Riley, S. (2009, March 10). Tech education will get some funding from stimulus effort. Investor's Business Daily Inc .
Rogers, C., Jarvin, L., & Barnett, G. (n.d.). Transforming elementary science through LEGO™ engineering design.
Sanders, M. (2009) STEM, STEM education, STEMmania. The Technology Teacher, 68 (4). 20-26.
Sanders, M. (1997). On research. Journal of Industrial Teacher Education, 24 (4), 57-59.
Shields, C. (2006). Engineering our future New Jersey. Proceedings of the 2006 Mid-Atlantic Section Conference of the American Society for Engineering Education , Toms River, New Jersey.
Snyder, J. F., & Hales, J. A. (Eds.). (1981). Jackson's Mill industrial arts curriculum theory . Reston, VA: International Technology Education Association.
Wicklein, R. C. (2006). Five good reason for engineering design as the focus for technology education. The Technology Teacher, 65 (7), 25-29.
Zuga, K. F. (2000). Some thoughts on technology education research . Presented at the American Association for the Advancement of Science, Conference on Research in Technology Education, Washington, DC.
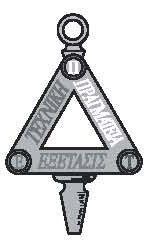