Advancing the "E" in K-12 STEM Education
Ronald Rockland, Diane S. Bloom, John Carpinelli, Levelle Burr-Alexander, Linda S. Hirsch and Howard Kimmel
Abstract
Technological fields, like engineering, are in desperate need of more qualified workers, yet not enough students are pursuing studies in science, technology, engineering, or mathematics (STEM) that would prepare them for technical careers. Unfortunately, many students have no interest in STEM careers, particularly engineering, because they are not exposed to topics in these fields during their K-12 studies. Most K-12 teachers have not been trained to integrate relevant STEM topics into their classroom teaching and curriculum materials. This article explores best practices for bringing engineering into the science and mathematics curriculum of secondary school classrooms by describing a project that utilizes concepts representing the merger of medicine, robotics, and information technology. Specific examples demonstrating the integration into the teaching of physics, biology, and chemistry are provided. Also considered are the critical issues of professional development for classroom teachers, improved preparation of future teachers of STEM, and the development of curriculum materials that address state and national content standards.
Introduction
Not enough students are interested in pursuing careers in science, mathematics, technology and especially engineering, at a time when the United States currently has a shortage of qualified workers in STEM fields ( NSB, 2008 ). One of the more critical reasons most students are not interested in pursuing careers in these fields is that they are not exposed to relevant topics in STEM, particularly engineering, during their K-12 studies. Quality curricular materials in these areas are scarce and teachers have not been trained to incorporate these topics into their curriculum and instruction ( Kimmel, Carpinelli, Burr-Alexander, & Rockland, 2006 ). Therefore, students are not adequately prepared to enter STEM programs in college or pursue careers in STEM fields ( NSB, 2008 ). As a result, there has been a growing interest in higher education to bring engineering principles and applications to secondary school mathematics and science classrooms ( Kimmel & Rockland, 2002 ; Kimmel, Carpinelli, Burr-Alexander, & Rockland, 2006 ). The integration of engineering concepts and applications into the different content areas in the curriculum is one approach. The engineering design process can provide a context that would support teachers in teaching about scientific inquiry since these processes are parallel in nature and have similar problem-solving characteristics.
Robotics encompasses the diverse areas of technology, computer science, engineering, and the sciences. Because of its multidisciplinary nature, using robotics in the classroom can be a valuable tool to increase student motivation and learning. The use of practical, hands-on applications of mathematical and scientific concepts across various engineering topics will help students to link scientific concepts with technology, problem solving, and design, and to apply their classroom lessons to real-life problems.
Teachers require a certain set of skills and knowledge to begin integrating technology and engineering concepts into their classroom practices ( Boettcher, Carlson, Cyr, & Shambhang, 2005 ; Zarske, Sullivan, Carlson, & Yowell, 2004 ). For new teachers this can be part of their pre-service training, but for current teachers comprehensive professional development programs are needed. Some identified factors that should be included in successful professional development programs include: long-term effort, technical assistance, and support networks, collegial atmosphere in which teachers share views and experiences, opportunities for reflection on one's own practice, focus on teaching for understanding through personal learning experiences, and professional development grounded in classroom practice.
This article provides a brief account of efforts to address the aforementioned issues and summarizes work that has been conducted at the New Jersey Institute of Technology to develop K-12 STEM curricular materials and training programs for secondary science and mathematics teachers in order to integrate engineering principles into classroom instruction.
Integrating Engineering into the Content Areas of Science
Many higher educational institutions have been working to bring technology and engineering principles into secondary school classrooms. The integration of engineering concepts into science and mathematics curriculum through interesting but practical applications helps increase students' interest in STEM, and it connects classroom lessons to the real world. Curriculum development and instructional strategies to support teachers in these efforts have been developed over the past decade ( Baker, Yasar, Kurplus,.Krause, & Roberts, 2004 ; Beven & Raudebaugh, 2004 ; Contrell, Pekcan, Itani,. & Velasquez-Brant, 2006 ; Harwood & Rudnistsky, 2005 ; Kimmel & Rockland, 2002 ; Poole, deGrazia, & Sullivan, 2001 ).
Currently, available curriculum materials need to create connections between the science used in engineering applications of the real world and science curriculum standards for which teachers and administrators are held accountable. Science can be viewed as proposing explanations for questions about the natural world, whereas engineering proposes solutions for problems of human adaptation to the real world. Instruction can emphasize the interdependence of these two disciplines as well as clarify their differences. The integration of engineering principles into science instruction, presented through problem-solving inquiry/discovery pedagogy, can stimulate students as well as enable them to recognize links between their lessons and tasks performed by engineers in the real world ( Harwood & Rudnistsky, 2005 ). When engineering and science are taught in tandem, they extend and reinforce each other.
Although curriculum materials and instructional strategies are necessary, they alone are not sufficient. What is also needed is effective professional development training for current teachers ( Kimmel, et. al., 2006 ; Zarske, et. al., 2004 ). Adequate new and pre-service teacher preparation programs ( Jones & Wang, 2001 ), that recognize the pressure on teachers to align their instruction with state content standards needs to be addressed as well ( Anderson-Roland et al., 2002 ; Fadali & Robinson, 1999 ; Loepp, 2004 ; Olds, Patel, Yalvac, Kanter, & Goel, 2004 ; Schaefer, Sullivan, & Yowell, 2003 ). Anderson-Roland et al. (2002) examined these issues and concluded that the system of education as well as the pressure to implement academic content standards and associated high-stakes state-wide assessments, were barriers to the degree by which science instruction and the curriculum can be changed or modified.
The Engineering Design Process and the Process of Scientific Inquiry
"Reasoning scientifically" or "thinking like a scientist" are two expressions frequently used by educators to describe the inquiry approach to teaching science. This instructional approach is reflected in the recommendations of the National Science Education Standards ( NRC, 1996 ) as a way to introduce students to how scientists actually conduct scientific inquiry. The reasoning and thinking aspects of the scientific inquiry process encompass learning outcomes such as understanding what it means to "know" something, understanding where knowledge comes from, being able to evaluate the validity of a knowledge claim, and understanding why knowledge is never final. Despite the importance of scientific thinking, it continues to be an elusive educational outcome for students, who do not seem to grasp how scientific theories arise and the manner in which evidence is used to support or call those theories into question. Many students do not understand the inquiry process because of the way it is taught, that is, the scientific method is a recipe for a step-by-step process that scientists follow to make discoveries.
Like scientists, engineers look at and think about the real world and what counts as knowledge. The engineering design process provides a context that can support teachers in the teaching of inquiry and scientific reasoning. It helps to bridge the disciplinary boundaries between science and engineering by drawing on engineering and engineering education as well as "mainstream" science as sources of ideas for instruction ( Lewis, 2006 ).
The thinking and problem-solving characteristic of the engineering design process is parallel to the scientific inquiry process ( Barak & Zadok, 2009 ; Lewis, 2006 ). Both processes focus on how a person knows things, the strength of that knowledge, and how that knowledge is related to evidence. Engineers are constantly making judgments about design, materials, and underlying theory as they engage in problem solving. Because engineers recognize that solutions to problems are only as good as the knowledge that supports them, and that sometimes solutions must be offered with incomplete knowledge, they view making constant knowledge improvement central to their work. The engineering design process is compared with the scientific inquiry process in Table 1 ( Harwood & Rudnitsky, 2005 ; Lewis, 2006 ).
Engineering Design | Scientific Inquiry |
---|---|
1. Identify the need or problem | 1. Formulate the problem |
2. Research the need of problem | 2. Information gathering |
3. Develop possible solutions | 3. Make hypotheses |
4. Select the best possible solution | 4. Plan the solution |
5. Construct a prototype | 5. Test solutions (perform experiments) |
6. Test and evaluate the solution | 6. Interpret data, Draw conclusions |
7. Communicate the solution | 7. Presentation of results |
8. Redesign | 8. Develop new hypotheses |
Asking the right questions and answering them in the most coherent manner is at the heart of both processes. For the engineer, constructing prototypes in order to make decisions bears a close resemblance to the experimentation of the scientist; both are aimed at answering questions. Incorporating engineering principles and design concepts into science curricula in ways that meet national and state science standards requires new knowledge and changes in classroom instruction.
Professional Development
Professional development for teachers is considered a key vehicle for educational reform and the need to change and improve classroom instructional practice ( Gibbons, Kimmel, & O'Shea, 1997 ; Guskey, 1986 ; Sparks, 1983 ). Professional development for teachers should introduce them to technological content and resources that expand their knowledge and their ability to apply new knowledge in the classroom A long-term professional development program that exposes science teachers to engineering principles and design can lead to the infusion of engineering principles and design into existing science classes that can be continued year after year and last through and beyond the training period ( Zarske et al., 2004 ).
Some of the key factors identified for effective professional development include: engaging teachers in practicing concrete tasks related to teaching, assessment, and observation of learning; drawing upon teachers' questions, inquiry, and experiences; including time for collaboration, sharing and exchange of ideas and practices; building on teachers' current work with students; and providing modeling, coaching, and problem-solving around specific areas of practice.
The planning of professional development programs that effectively lead to desired teaching practices is not a simple process. Too often, short-term training institutes and after school workshops are seen as ends in themselves. "One-shot" approaches to staff development fail to produce lasting changes in teachers' behavior because these teachers are not provided with the opportunity to experience success. Staff development efforts often focus on isolated instructional behaviors, such as cooperative learning, teaching to learning styles, or classroom management skills.
An alternative perspective on the features influencing effective professional development outcomes is provided by Yoon and colleagues ( Yoon, Garet, Birman, & Jacobson (2006) , who consider five features and three core features follow: (1) Active Learning: Teachers are involved in discussion, planning, and practice, (2) Coherence: Activities are built on what they are learning and lead to more advanced work, (3) Content Focus: Content is designed to improve and enhance teachers' knowledge and skills, and two structural features follow: (1) Duration: Professional development for teachers extend over a two-year period, and (2) Collective Participation: Teachers meet in discipline and grade level groups to discuss strategies and content, and to develop approaches that they present to their peers.
Prior Efforts at NJIT
The Pre-Engineering Instructional and Outreach Program (PrE-IOP) at the New Jersey Institute of Technology's Center for Pre-College Programs was established to inform students, teachers, parents, and school counselors about careers in engineering and to provide teachers with pre-engineering modules that could be integrated into their secondary school science curricula, along with professional development on content and instructional strategies for the modules ( Kimmel & Rockland, 2002 ; Carpinelli, Burr-Alexander, Hanesian, Kimmel, &Sodhi, 2004 ). The modules were developed to incorporate multiple engineering disciplines including biomedical, chemical ( Hanesian, Burr-Alexander, Kimmel, Kisutcza, & Tomkins, 2004 ), civil, electrical and mechanical, all appropriate for integration into life and physical sciences. Survey instruments were developed to measure students' ( Gibbons, et al., 2004 ; Hirsch, Gibbons, Kimmel, Rockland, & Bloom, 2003 ), teachers' ( Hirsch, Kimmel, Rockland, & Bloom, 2005 ), parents' and school counselors' ( Gibbons, et. al., 2003 ) attitudes engineering and knowledge about engineering careers to help evaluate the impact of the pre-engineering curriculum and the effectiveness of the PrE-IOP training programs. Teachers' attitudes and knowledge about engineering careers, their concerns about implementing the curricula, along with their self-reported preparedness to teach the new modules were examined longitudinally across two academic years. Repeated measures of analysis of variance found significant increases across the two years ( Hirsch, Kimmel, Rockland, & Bloom, 2006 ). Students' attitudes engineering and knowledge about engineering careers were also examined and significant increases were found during the school year following teachers' training. The attitudes and knowledge about engineering careers for students taught by the same teachers during the second year after training were significantly higher than for students taught by the teachers' colleagues who taught the same classes but did not participate in the training program ( Hirsch, et. al., 2006 ).
Medibotics: The Merging of Medicine, Robotics, and Information Technology
Because of its multidisciplinary nature, the study of robotics in the classroom can be a valuable tool for the practical, hands-on application of concepts across various engineering and science topics ( Beer, Chiel, & Drushel, 1999 ; Eguchi, 2009 ). The Medibotics project was developed to use robotics as a teaching tool to increase student motivation to utilize information technology (IT) applications to learn scientific and mathematical concepts, and to link them to technology, problem solving, and design. Medibotics represents the merging of the specialties of medicine, robotics, and information technology, as it focuses on the development of projects that are medical in origin. The curriculum developed for Medibotics enables the incorporation of IT, engineering, and robotics into the science and mathematics curricula of secondary school classrooms by teaching students to design and build robots to perform simulated computer-assisted surgeries.
A professional development program aligned with the factors described by Yoon and colleagues (2006) was designed to train teachers to use the Medibotics curriculum. The program consisted of an initial two-week summer workshop, a one-week summer workshop the following summer, and an academic year follow-up that included one-day workshops and in-class support by university faculty, staff, and graduate students during their implementation process in the classroom. In addition, an electronic peer-learning community was established for communications among teachers and university personnel and for online professional development activities.
The Medibotics curriculum used LEGO™ MINDSTORMS for Schools with ROBOLAB programming software kits to solve biomedical engineering problems. The ROBOLAB software uses an icon-based, diagram-building environment to write programs, and it is based on LabVIEW™, from National Instruments, the most popular software used in biomedical engineering. This icon-based environment enables students at lower grades to perform simple to complex programming tasks. Using these types of kits to teach students to build robots provides an overview of how multiple fields of science, such as biology, medicine, engineering (sensors and motors), and physics (gears, shifts, belts, wheels, axles and hinges) can be combined with information technology (the programming languages that help support the input and output from sensors to motors) to solve real-world problems. In order to move and control their robots, students must become familiar with basic actuators, some basic motor controls, and the use of sensors to provide feedback of position.
The simulated robotic surgeries have elements of actual medical procedures. Each surgery entails a different set of tasks and sequence of actions, requiring the development of different procedures and programs using the LEGO™ MINDSTORMS for Schools with ROBOLAB programming software. The robotic surgeries have been developed to demonstrate various surgical procedures and physiological topics; to demonstrate physical forces and design principles; to utilize various sensors, and relate scientific principles to the sensors; to use common food or craft products that are inexpensive and easy to obtain and maintain (avoiding meat and nut products that need refrigeration); to enable students to understand basic programming concepts; and to demonstrate complex programming in which the robot has to perform actions based its sensors.
One teacher from the Medibotics program augmented a life science lesson with examples of how robots could be used to enhance the study of functioning parts of the skeletal system. Comparisons were made between the joints of humans and robots and their comparative flexibility. Students experienced both types of structure and how each performed based on its assets and limitations. Conversation with the instructor indicated that he planned to coordinate a robotic experience with each of the content areas in the school curriculum. Another teacher modified the ninth grade general science curriculum, so that robotics had been integrated into each of the areas of earth, physical, and life science. For example, in earth science, students programmed robots to explore the surface of Mars. Robotic surgery was introduced as an application in the life sciences.
Robotics as a Vehicle to Bring Engineering Concepts into the Science Classroom
Robotics provides many opportunities to use engineering and information technology to enhance science instruction for both the teacher and the students. The integration of robotics into the science curriculum capitalizes on the embedded science concepts ( Kimmel, Carpinelli, Burr-Alexander, Hirsch, & Rockland, 2008 ; Chambers, Carbonaro, & Rex, 2008 ). The design, construction, and control of the robots by the students contribute to the learners' acquisition of knowledge and the refinement of their thinking skills regarding scientific, engineering design, and information technology. The robotic surgeries provide teachers with the opportunity to move the study of scientific concepts from the textbook to hands-on learning of biology topics, such as anatomy and physiology, and chemistry topics, such as acids and bases.
The construction and operation of the robot itself demonstrates applied physical concepts including motion of objects, levers, gears, forces, rotational torque, movement of the robotic arm (mechanics), principles of electricity and basic circuitry. Statements of expectations of students, related to these topics are provided in the State Content Standards ( NJDOE, 2008 ). For example, Standard 5.7 (Physics): All Students Will Gain an Understanding of Natural Laws as They Apply To Motion, Forces, And Energy Transformations:
- When more than one force acts on an object at the same time, the forces can reinforce or cancel each other producing a net force that will change speed and/or direction of the object.
- Whenever one object exerts a force on another, an equal and opposite force is exerted on the first object.
While principles of applied physics would apply to any of the robotic surgeries, incorporating the Medibotics curricula into the life sciences, chemistry, and physics would depend on the specific surgery and the choice of sensor. Understanding energy including light, heat, sound, electricity, and magnetism is necessary for the use of sensors, such as the light sensor and the sound sensor, and requires knowledge of properties of light and optics, transfer of energy, and waves. Student expectations related to these topics are provided in the State Content Standards ( NJDOE, 2008 ). For example, Standard 5.7 (Physics): All Students Will Gain An Understanding Of Natural Laws As They Apply To Motion, Forces, And Energy Transformations.
- Describe the nature of various forms of energy, including heat, light, sound, mechanical, and electrical energy transformations from one form to another.
- Explain how the various forms of energy (heat, electricity, sound, light) move through materials and identify the factors that affect that movement.
A sound sensor is essentially a microphone which is a type of transducer; an electrical component that will take a mechanical input and turn it into an electrical one. The mechanical input in this particular sensor is the pressure from sound that causes a small plate to vibrate. A light sensor is a sensor responsible for giving the robot a visible sense of its surroundings. The sensor has two main roles; the first is to gather a reading of the ambient light in the sensor's surroundings and the second is to generate a beam of light and gather a reading from the light reflected off of the surface. A color sensor doesn't measure the intensity of white light; instead, a color sensor measures the intensity of red, blue, and green light. The light sensor can only distinguish between one or two different shades of color, whereas a color sensor can distinguish each individual color, allowing one sensor to identify several different objects by color.
The robotic surgeries also can be used in biology to teach physiology and anatomy. Statements of expectations of students related to these topics are provided in the State Content Standards ( NJDOE, 2008 ). For example, Standard 5.5 (Characteristics of Life): All Students Will Gain an Understanding of The Structure, Characteristics, And Basic Needs of Organisms and Will Investigate the Diversity of Life.
- Describe the basic functions of the major systems of the human body including, but not limited to, the digestive, circulatory, respiratory, skeletal, and muscular systems.
- Explain how systems of the human body are interrelated and regulate the body's internal environment.
- Recognize that complex multicellular organisms, including humans, are composed of and defined by interactions of tissues, organs, and systems.
One surgery simulates a heart bypass operation. From this surgery, students can learn about coronary circulation and how the coronary circulation consists of the blood vessels that supply blood to and from the heart muscle. They can also learn about the two main coronary arteries. Good heart function means that the heart will function properly. Coronary disease occurs when these relatively narrow vessels are commonly affected by a build up of plaque. As plaque builds up, the coronary arteries become narrow and stiff. Blood flow to the heart is reduced. This lack of blood flow decreases the oxygen supply to the heart muscle. The result is either angina or a heart attack. The heart bypass surgery uses "twizzlers" to represent blood vessels, the red (healthy), and the black (unhealthy). The robot is designed to perform the corresponding surgery that would move the robot to the "blood vessel", test it, and if it is healthy (red), leave it alone. If the "blood vessel" is unhealthy (black), the robot should remove it and replace it with a healthy one. Students can also discuss what happens to other systems in the body if the circulatory system performs inadequately because the heart cannot pump efficiently
Another surgery illustrates the biological principles in Fundoplication, the standard surgical method to treat gastro-esophageal reflux disease (GERD), which causes inflammation, pain (heartburn), or other serious complications. GERD causes acid to come back up from the stomach into the esophagus. Fundoplication is the surgical technique that strengthens the barrier to acid reflux by closing off the esophagus from the stomach. This surgery requires that students are able to identify and describe the functions of the major components of the digestive system, stomach, esophagus and that they learn about stomach acid; breakdown of food to pull out nutrients and energy; acid-reflux disease; and medical justifications for surgical/non-surgical treatments.
Fundoplication can also be used in chemistry so students can learn about acids and bases, the pH scale, neutralization of acids, and function of antacids. Students have the opportunity to create a mock pH scale using the color sensor, and then use it to identify the pH of the stomach acid. Experiments can be carried out to determine the effects of antacids on pH by creating a graph depicting experimental results as well as explaining which antacid had the most desirable effect. Statements of expectations of students, related to these topics are provided in the State Content Standards ( NJDOE, 2008 ). For example, Standard 5.6 (Chemistry): All Students Will Gain an Understanding of The Structure and Behavior of Matter.
- Describe the properties of mixtures and solutions, including concentration and saturation.
- Show how substances can chemically react with each other to form new substances having properties different from those of the original substances.
Engineering in Teacher Preparation Programs
Increasing the presence of engineering in the K-12 curriculum will require more qualified and better prepared math, science, and other discipline teachers. The education of science and mathematics teachers in different content areas generally does not include courses that promote an understanding of engineering principles and design. Bringing engineering into K-12 classrooms will require modifications of programs for teachers of science and mathematics. This can be addressed by exposing teachers to preservice training on engineering concepts that show them how they can integrate these concepts into the classroom. The approach that is most realistic "is to blend engineering concepts and exercises into math, science, and other classes in elementary, middle, and high schools" ( Cavanagh, 2009 , para. 11).
The training of K-12 teachers requires a viable plan that will allow more students to be prepared for careers in engineering. This is accomplished by preparing teachers to bring engineering into their K-12 classrooms by addressing five key factors: (1) Instruction; (2) Student Learning; (3) Time; (4) Resources; and (5) Training.
A framework for pre-service teachers to blend engineering concepts is Preparation, Assistance, and Reflection (PAR) ( Richardson, Morgan, & Fleener, 2009 ), an instructional strategy that can be used to focus instruction on the learner and learner outcomes. Learners' prior knowledge and interest are critical to integrating effective engineering concepts into the curriculum. There are many ways to prepare, assist, and extend students' understanding and application of engineering learning objectives. Although PAR was developed to help teach students to read and comprehend text structures across all disciplines, herein it is considered applicable to an integrated, student-engaged and interconnected K-12 educational system for teaching engineering. Engineering lessons on how products are designed and built fit well with science, technology, and math education at the K-12 level. Students who live in the 21st century are immersed in "a world that's shaped by engineering," while in the early 1990s, "almost no engineering curricula or programs existed" ( Cavanagh, 2009 , para. 8). Unlike mathematics and science, engineering has no formal standards or assessment measures of student learning. The What and How of learning across the stages of PAR imparts for both teacher and student, the vibrant exchange of the learning cycle. David Small, Pentagon mechanical engineer on missile defense system states that "a key element in designing a 'pre-engineering' or engineering course, as well as teaching it, is always keeping in mind where the students are, both in life and in the learning process" ( Aronowitz, 2009 , para. 10).
The What of the student learning cycle are the K-12 national and state learning objectives of mathematics, science, and technology that accompany the requisite assessment measures that parallel the key trends and issues in engineering education, while the How is the interest-based engineering activities that encourage learning through PAR. The How based activities are age-, grade- and interest-appropriate hands-on learning activities that address engineering concepts through problem-based tasks that include conception (P), development (A), and the building (R) of technological gadgets, such as transistor radios, burglar alarms, electronic timers, telephones, cameras, computers, robots, bridges, go-carts, theme park rides, and roller coasters. The focus for exposing K-12 students to engineering, as it does in other successful learning endeavors, must be on student interest ( Daly, 2008 ). Pre-service teachers need to be given the opportunity to learn how to adopt, adapt, and/or develop interest-appropriate hands-on learning activities. This is what and how lessons leap to life as Michele Miller writes in her article about fifth grade teacher Annie McCallister who developed 45-minute lab activities using simple inexpensive supplies from recycling centers and home building supply centers to capture teaching time and student interest by having students "interact with other things besides a book" ( Miller, 2010 , "New Science Lab," para. 7). McCallister makes her point by using her guitar to teach a lesson on sound energy. In addition, pipe insulation from a home supply store is used by students to make miniature roller coasters to understand physics. According to Samantha Murray, K-12 coordinator for the American Society for Engineering Education (ASEE), targeting middle school and high school students requires a "vigorous" integrated curriculum that creates a natural progression for learning "by getting students in the mindset of being able to think about engineering as a possibility" ( McCrea, 2009 , para. 6) and a career "long before it comes time to select a major" ( McCrea, 2009 , para. 8). The mindset can be created if educators pursue student interests while teaching and exposing students to take a product apart and put it back together or, according to Savannah, Georgia, high school educator David Small, achieve the like using "a CAD [tool] to sketch a 2D world and then manipulate it to create a 3D figure to manipulate it [onscreen] depending on what you're trying to develop" ( Aronowitz, 2009 , "Assembly, the Right Components," para. 17).
Knowing what and how students learn and to engage them as active learners, one must take into account the teacher who is the not the "sage on the stage," but, the "guide on the side" ( King, 1993 , p. 30). To successfully accomplish this learning cycle the teacher must address how students learn by acknowledging and practicing research findings from National Training Laboratories in Bethel, Maine, on types of instruction versus long-term retention ( Haun, 2002 ). This level of learning, resulting in retention and transfer, occurs most efficiently through concrete activity-based experiences. Active learning involves input from multiple sources through multiple senses (hearing, seeing, feeling, etc.). The Learning Pyramid presents that students learn and retain 90% of new knowledge when they are engaged in purposeful activities with their peers, that is, students teach others and students immediately use and apply new information. In contrast, only 5% is learned and retained when teachers use lecture and 10% is retained when students read to themselves and use the textbook to present and learn new knowledge. It has also been stated that there is a need for core concepts and ideas with less of an emphasis on scientific steps on how to make a device or establish a process specific to a task ( Cavanaugh, 2009 , para. 9).
In K-12 schools the focus has unfortunately been on the topic "engineering design" at the neglect of engineering principles and processes with hands-on applications. Cavanaugh (2009) further suggests that K-12 teachers and school programs should be designed so engineering lessons ". . . ask students to make use of math, science, and technology knowledge and skills. . . and emphasize problem solving, the ability to use equipment and technology, communication and collaboration with others" (para. 10). Not withstanding that teachers are governed by the constraints of time and delivery for the courses they teach, there are governing components of teaching, that is, the strategies and techniques of the what and how of the discipline. According to Small, what makes "a good fit for the course is that even though professional engineers use it to design much more advanced components, the basic application is designed for broad use, from beginners to trained technicians to technology manufacturing professionals. . . like a calculator, its functions can be applied at all levels of education and productivity" ( Aronowitz, 2009 , para. 16). The what and how of learning across the preparation, assistance, and reflection stages of teaching imparts the exchange of learning for both the teacher and the student. Within the teaching and learning cycle both the teacher and student are accountable and reflective of what is learned and how as it relates to learning, application, and extension of this knowledge to new and varied settings that gives students, according to Small, "a practical idea of what may be required of them in pursuing a career they had previously decided they wanted based solely on an abstract concept of what it entailed" ( Aronowitz, 2009 , "Not Engineers Yet," para. 19). Roger Yancey, Headmaster of Savanna Christian Preparatory School, finds "this awareness in high school as a way of narrowing students' focus once they're in college and making long-term decisions". . . and research at Armstrong University in Savannah shows at least it reduces the number of 'change of majors' before graduating" ( Aronowitz, 2009 , "Not Engineers Yet," para. 20).
The key to success is assessment integrally tied to the learner and outcomes. Outcomes stated and modeled on the Preparation stage of learning and focused on a static, nonmoving target enable students to focus clearly on learning to comprehend new knowledge, thereby moving seamlessly through knowledge, comprehension, application, analysis, synthesis, and evaluation of Bloom's taxonomy of learning. The features of PAR allow students to (P) encounter new knowledge (discovered or presented), (A) have knowledge modeled with an opportunity to practice in order to verify success or, if not success, correct learned behavior by being re-taught and allowed to practice again the new knowledge, until learning has been achieved to the level of expectation for the individual and in keeping with the objective and the identified assessment measure. Additional practice of new knowledge in similar and/or diverse settings during the (R) reflection stage stabilizes the learner's intake of the new concept while the assessment, directly linked to that which occurred in the PAR phase, ensures the student and the teacher that learning has taken place. Additional exposure of the concept affords retention if the teacher revisits the concept learned in strategically planned, intermittent occurrences over ensuing 2-3 week periods. The value of the assessment to both the learner and teacher is directly related to the learning objectives and the teacher's what and how of delivery. If students learn successfully, the assessment measure validates the learning, if not, and the measure has fidelity to the course of objectives, then the teacher needs to re-teach using a different or revised set of protocols for instructional delivery. In any case, during preservice education, teachers need to learn how to reflect on their instructional decision-making, evaluate their teaching performance, and adapt their performances to ensure learners' success, and thus teachers' success. In our increasingly technological and knowledge-based competitive global society, it is critical to produce more engineers in the United States and to increase awareness about engineering in order to support and use engineering for a more efficient, effective, safe, and secure world community. The application of PAR is one framework for integrating engineering education into the K-12 curriculum.
Summary
This article contributes to the dialogue that explores best practices for bringing engineering principles and applications into the science and mathematics curricula. Areas of consideration include the development of curriculum materials and instructional strategies for classroom teachers; effective professional development for the current teachers; effective alternative preparation of new teachers; and using engineering topics to achieve state and national content standards. Prior efforts to establish effective professional development for teachers of science and mathematics are discussed. Successful programs that include specific examples have been described and should serve as models for others. Implementation of successful models should lead to a future workforce that is more technologically literate, and that ultimately includes more engineers to meet the challenges of the coming years.
Dr. Ronald Rockland is a Professor of Engineering Technology and Biomedical Engineering, and Chair of the Department of Engineering Technology at New Jersey Institute of Technology, Newark
Dr. Diane S. Bloom is an Assistant Professor in the Department of Elementary Education and Bilingual Education at Kean University, Union, New Jersey.
Dr. John Carpinelli is a Professor of Electrical and Computer Engineering at New Jersey Institute of Technology, Newark.
Ms. Levelle Burr-Alexander is a senior member of the professional staff at Center for Pre-College Programs at New Jersey Institute of Technology, Newark .
Dr. Linda S. Hirsch is a senior member of the professional staff at the Center for Pre-College Programs at New Jersey Institute of Technology, Newark.
Dr. Howard Kimmel is a professor of Chemical Engineering at New Jersey Institute of Technology, Newark, and Executive Director of its Center for Pre-College Programs.
References
Anagnostopoulos, C., Gorham, D., Newberry, P., Stoler, B., & Sechrist, C. (2001). Pre-college engineering education and standards for technological literacy. Proceedings of the 2001 ASEE Annual Conference , Albuquerque, NM.
Anderson-Rowland, M., Baker, D. R., Secola, P. M., Smiley, B. A., Evans, D. L., & Middleton, J. A. (2002). Integrating engineering concepts under current K-12 state and national standards. Proceedings of the 2002 ASEE Annual Conference , Montreal, PQ, Canada.
Aronowitz, S. (2009, June 11). Re: Engineering in the 21st century. THE Journal , Retrieved from. http://thejournal.com/articles/2009/06/11/engineering-21st-century-skills.aspx
Baker, D. R., Yasar, S., Kurplus, S. E. R., Krause, S. J., & Roberts, C. (2004). Not just for Nerds: Embedding sience activities with a design, engineering, and technology environment. Proceedings of the 34th ASEE/IEEE Frontiers in Education Conference , Savannah, GA.
Barak, M. & Zadek, Y. (2009). Robotics projects and learning concepts in science, technology and problem solving. International Journal of Technology & Design Education, 19 , 289-307.
Beer, R. D., Chiel, H. J., & Drushel, R. F. (1999). Using autonomous robotics to teach science and engineering. Communications of the ACM, 42 (6), 85-92.
Beven, R. Q., & Raudebaugh, R. A. (2004). A model for unified science and technology. The Journal of Technology Studies, 30 (1), 10-15.
Boettcher, B., Carlson, B., Cyr, M., & Shambhang, S. (2005). Engineering implementation in grades 6-12: Evaluation of the effectiveness of a workshop model. Proceedings of the 35th ASEE/IEEE Frontiers in Education Conference , Portland, OR.
Carpinelli, J., Burr-Alexander, L., Hanesian, D., Kimmel, H., & Sodhi, R. (2004) The pre-engineering instructional and outreach program at the New Jersey Institute of Technology. Proceedings of the International Conference on Engineering Education , Gainesville, FL.
Cavanagh, S. (2009, September 8). Panel wants engineering integrated into the curriculum. Education Week. Retrieved from http://www.edweek.org/ew/articles/2009/09/08/03engineering.h29.html
Chambers, J. M., Carbonaro, M., & Rex, M. (2007). Scaffolding knowledge construction through robotic technology: A middle school case study. Electronic Journal for the Integration of Technology in Education, 6 , 55-70.
Contrell, P., Pekcan, G., Itani, A., & Velasquez-Brant, N. (2006). The effects of engineering modules on student learning in middle school science classrooms. Journal of Engineering Education, 95 , 301-309.
Daly, T. (2008). Fly under the radar, soar as a teacher. Special Report:7 Days to a Perfect Classroom . San Diego, CA: Smartypants Publications.
Eguchi, A. (2009). What are students learning from educational robotics? Different approaches to educational robotics. Proceedings of 20th International SITE Conference , Charleston, SC.
Fadali, M. S., & Robinson, M. (1999). How do the National Science Education Standards support the teaching of engineering principles and design? Proceedings of the 30th ASEE/IEEE Frontiers in Education Conference , Kansas City, MO.
Gibbons, S., Kimmel, H., & O'Shea, M. (1997). Changing teacher behavior through staff development: Implementing the teaching and content standards in science. School Science and Mathematics, 97 (6), 302-309.
Gibbons, S. J., Hirsch, L. S., Kimmel, H., Rockland, R., & Bloom, J. (2003). Counselors attitudes and knowledge about engineering. Proceedings of the 2003 International Conference on Engineering Education , Valencia, Spain.
Gibbons, S. J., Hirsch, L. S., Kimmel, H., Rockland, R., & Bloom, J. (2004, October). Middle school students' attitudes to and knowledge about engineering. Proceedings of the International Conference on Engineering Education , Gainesville, FL.
Guskey, T. R. (1986). Staff development and the process of teacher change. Educational Researcher, 15 , 5-12.
Hanesian, D., Burr-Alexander, L., Kimmel, H., Kisutcza, J., & Tomkins, R. P. T. (2004). Integrating chemical engineering as a vehicle to enhance high school science instruction. Proceedings of the 2004 ASEE Annual Conference , Salt Lake City, UT.
Harwood, J., & Rudnitsky, A. (2005). Learning about scientific inquiry through engineering. Proceedings of the 2005 ASEE Annual Conference , Portland, OR.
Haun, P. (2002). Active learning online learning pyramid. Bethel, Maine: National Training Laboratories.
Hirsch, L. S., Gibbons, S. J., Kimmel, H., Rockland, R., & Bloom, J. (2003, November). High school students' attitudes to and knowledge about engineering. Proceedings of the 33rd ASEE/IEEE Frontiers in Education Conference , Boulder, CO.
Hirsch, L .S., Kimmel, H., Rockland, R., & Bloom, J. (2005). Implementing pre-engineering curricula in high school science and mathematics. Proceedings of the 35th ASEE/IEEE Frontiers in Education Conference , Indianapolis, IN.
Hirsch, L. S., Kimmel, H., Rockland, R., & Bloom, J. (2006). Using pre-engineering curricula in high school science and mathematics: A follow-up study. Proceedings of the 36nd ASEE/IEEE Frontiers in Education Conference , San Diego, CA.
Jones, R., & Wang, E. (2001). Experiences in an engineering technology course for education majors. Proceedings of the 31st ASEE/IEEE Frontiers in Education Conference , Reno, NV.
Kimmel, H., & Rockland, R. (2002). Incorporating pre-engineering lessons into secondary science classrooms. Proceedings of the 32nd ASEE/IEEE Frontiers in Education Conference , Boston, MA.
Kimmel, H., Carpinelli, J., Burr-Alexander, & L. Rockland, R. (2006). Bringing engineering into K-12 schools: Problem looking for solutions? Proceedings of the 2006 ASEE Annual Conference , Chicago, IL.
Kimmel, H., Carpinelli, J., Burr-Alexander, L, Hirsch, L. S., & Rockland, R. (2008). Introducing robotics into the secondary science classrooms. Proceedings of the 19th International SITE Conference , Las Vegas, NV.
King, A. (1993). From sage on the stage to guide on the sde. College Teaching, 41 (1), 30-35. Retrieved from http://www.questia.com/PM.qst?a=o&d=94305197
Lewis, T. (2006). Design and inquiry: Bases for an accommodation between science and technology education in the curriculum? Journal of Research in Science Teaching, 43 (3), 255-281.
Loepp, F. L. (2004). Standards: Mathematics and science compared to technological literacy. The Journal of Technology Studies, 30 (1), 2-10.
McCrea, B. (2009, March 12). Interview: Cultivating engineers in K-12. THE Journal . Retrieved from http://thejournal.com/articles/2009/03/12/interview-cultivating-engineers-in-k12.aspx
Miller, M. (2010, February 2). Where lessons leap with life. Retrieved from http://www.tampabay.com/news/education/k12/new-science-lab-at-mary-giella-elementary-brings lessons-to-life/1070095
National Research Council [NRC]. (1996). National science education standards . Washington, DC: National Academy Press.
National Science Board [NSB]. (2008). Science and engineering indicators 2008. Arlington, VA: National Science Foundation (NSB-08-1).
New Jersey Department of Education. [NJDOE]. (2008). Retrieved from http://www.state.nj.us/njded/cccs/
Olds, S., Patel, C., Yalvac, B., Kanter, D., & Goel, N. (2004). Developing a standards-based K-12 surricula through partnerships with university students and industry. Proceedings of the 2004 ASEE Annual Conference , Salt Lake City, UT.
Poole, S. J., deGrazia, J. L., & Sullivan, J. F. (2001). Assessing K-12 pre-engineering outreach programs. Journal of Engineering Education, 90 (1), 43-48.
Richardson, J. S., Morgan, R. F., & Fleener, C. E. (2009) Reading to learn in the content areas (7th ed.). Belmont, CA: Thompson Wadsworth Publications.
Schaefer, M., Sullivan, J., & Yowell, J. (2003). Standards-based engineering curricula as a vehicle for K-12 science and math integration. Proceedings of the 33rd ASEE/IEEE Frontiers in Education Conference, Boulder, CO.
Sparks, G. (1983). Synthesis of research on staff development for effective teaching. Educational Leadership, 41 (3), 65-72.
Yoon, K. S., Garet, M., Birman, B., & Jacobson, R. (2006). The effects of mathematics and science professional development on teachers' instructional practice: Using professional development activity log. Washington, DC: Council of Chief State School Officers.
Zarske, M., Sullivan, J., Carlson, L., & Yowell, J. (2004). Teachers teaching teachers: Linking K-12 engineering curricula with teacher professional development. Proceedings of the 2004 ASEE Annual Conference, Salt Lake City, UT.
Acknowledgments
The discussion of the Medibotics project is based upon work supported by a grant from the National Science Foundation under Grant No. 0624434. Any opinions, findings and conclusions or recommendations expressed in this material are those of the authors and do not necessarily reflect the views of the National Science Foundation (NSF).
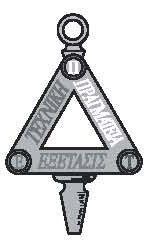