Engineering Efforts and Opportunities in the National Science Foundation's Math and Science Partnerships (MSP) Program
Engineering Efforts and Opportunities in the National Science Foundation's Math and Science Partnerships (MSP) Program
Pamela Brown and Maura Borrego
The National Science Foundation's Math and Science Partnership (MSP) program ( NSF, 2012 ) supports partnerships between K-12 school districts and institutions of higher education (IHEs) and has been funding projects to improve STEM education in K-12 since 2002. Some projects also include business/industry, informal science organizations, and State Departments of Education as partners ( NSF, 2008 ). As of 2011, a total of 178 MSP projects have received support as part of a STEM education investment of over $900 million. The MSP program has evolved as field-driven strategies and opportunities are created, NSF priorities change, and new national trends appear (e.g., the Common Core State Standards in Science and Mathematics). Indeed, the most recent set of guidelines for proposals (NSF Solicitation 12-518), released in December 2011, is scheduled to be updated again. The MSP program remains a major research and development effort to support innovative partnerships to improve K-12 student achievement in mathematics and science while conducting STEM education research. The current solicitation requests proposals for two levels of partnerships - implementation and prototype - concentrating on one of four focal areas: (a) community enterprise for STEM learning, (b) current issues related to STEM content, (c) identifying and cultivating exceptional talent, and (d) K-12 STEM teacher preparation.
One important movement over the past decade has been increasing interest in incorporating engineering and design content in K-12 teaching and learning, a strategy validated in the National Research Council report, "A Framework for K-12 Science Education: Practices, Crosscutting Concepts and Core Ideas" ( NRC, 2011 ). The goals of K-12 engineering and design content traditionally have been to prepare students to think critically, creatively, and independently by solving problems with real-world applications.
Engineering is gaining ground as a content area in the K-12 classroom. Numerous programs around the country, some of them quite large (e.g., Project Lead the Way, Infinity Project, Engineering is Elementary, Engineering by Design, Children Designing and Engineering), are developing and delivering curriculum and teacher education in engineering at the pre-college level. ( National Academy of Engineering, 2012 )
Additional benefits more recently identified are the potential for recruitment and better preparation of future engineering students. In an effort to explore how engineering and design are being implemented in MSP projects, we synthesize strategies and findings from the NSF MSP portfolio, including publicly available award information from http://nsf.gov and http://MSPnet.org . This descriptive analysis is supplemented by data from annual project surveys conducted by a contractor (Westat) on behalf of NSF. We report on the ways that engineering and design content are being implemented by MSP projects, along with associated challenges and opportunities.
Background and Literature Survey
MSP projects go beyond typical approaches to improving K-12 STEM education through inclusion of educational research as part of project design and intellectual engagement of higher education STEM faculty in K-12 reform. Individual projects differ in their activities and scope. For example, nearly 40% of partnership projects focus on math and nearly 30% on science. Of the remainder, many consider both mathematics and science, four projects focus uniquely on engineering education, and another group attempts to integrate engineering with science and/or mathematics. Of the schools involved in MSP, 45% are primarily elementary, 28% middle, and 27% high school level. Over 90% of projects conduct workshops, institutes, or courses with K-12 teachers that increase content and/or pedagogical knowledge while also developing and utilizing leadership skills. An additional promising mechanism used by far fewer partnerships was providing externship opportunities for teachers. One engineering-focused strategy for improving K-12 education is to introduce engaging engineering design and concepts to teachers in order to provide contemporary real-world examples. These interventions are based on the logic that if teachers are given enhanced professional development through increased content knowledge, model teaching practices, and authentic experiences in one or more of the STEM disciplines, that would impact how they teach, which would then ultimately impact the learning of students. The engineering content has the potential benefit to improve learning in mathematics and science by motivating students and developing their critical thinking and problem solving skills. A shared learning experience focused on relevant, real-world challenges is a proven strategy for fostering student learning of and engagement with mathematics and science ( Project Kaleidoscope, 2006 ).
Another potential benefit to engineering content in the K-12 curriculum, in addition to promotion of engineering awareness and literacy to better prepare engineering majors before starting college, is recruitment of engineering students. Personal interest has been shown to be a key factor in selection of a major. Input from parents, friends, relatives, professor/teachers, and counselors as well as beginning salary, earning potential, and opportunities for advancement are other factors ( Beggs, 2008; Kuechler, 2009 ). However, all of these factors require having knowledge of that major, and the majority of high school students are not currently introduced to engineering professions in K-12. Additionally, in a survey of high school parents, counselors, and science and mathematics high school teachers, their knowledge of STEM occupations was found to be limited, particularly in information technology and engineering ( Hall, 2011 ). Reaching out to high school students to recruit engineering students is critical to increasing the number of engineering graduates. Nationally, 93% of students enrolled in engineering after eight semesters began as freshmen with this same major. In other majors, the same major rate of retention ranged from just 35%-59% ( Ohland, 2008 ). While engineering has a high persistence rate compared to other majors, engineering majors are not attracting undeclared students or those transferring from other majors ( Ohland, 2008 ). An introduction to engaging engineering content prior to the start of college may pique personal interest and hence result in more freshmen selecting engineering majors.
From a pedagogical perspective, engineering is the link that ties together mathematics and science (Katehi, et al., 2009). The integrative, application focused nature of engineering can improve student learning and increase test scores, which helps schools satisfy standards-driven education requirements ( Baker, 2005 ; Silk, 2009 ; Custer, 2011 ). The use of engineering design provides practical classroom benefits for both educators and students. The collaborative, socially beneficial aspects of engineering have also been shown to appeal to students whom the field has traditionally failed to engage, including females and underrepresented minorities (Geddis, Onslow, Beynon, & Oesch, 1993; Wiest, 2004).
Methods
To explore how engineering and design are being implemented in MSP projects, we first searched the abstracts of all active and expired MSP projects (funded through 2011) for the term engineer. From this list we excluded any projects that only included engineering as an expansion of the acronym STEM (the sole reference to engineering was that the acronym STEM was written out-Science, Technology, Engineering, and Mathematics). This resulted in 31 projects for further analysis. For each, we examined the original proposal and most recent annual or final report, if available. If the managing program officer was available, we asked this person about engineering aspects of the project. The following are the questions we asked the program officers in an informal interview:
- Which of these projects do you recall having an engineering component?
- In what ways are engineering higher education faculty involved in the project(s)?
- What engineering content is involved, and at what grade levels?
- In what ways are preservice teachers being trained in engineering? Is teacher training the only way that undergraduates are involved in the project?
- In what ways are in-service teachers being trained in engineering?
- What unique challenges and opportunities do you see in incorporating engineering and engineering design into the K-12 curriculum, in order to improve STEM education?
We excluded cases in which engineering was initially included as part of a more general STEM approach but was not mentioned in subsequent work. For example, in one project a focus on energy turned out to be an examination of photosynthesis. This process resulted in the 17 projects listed in Table 1 that we found to include some aspect of engineering. A limitation of this approach is the subjective nature of what is and is not engineering. However, the two authors, both engineers, worked together to develop and apply a consistent definition- projects which included engineering content.
Results and Discussion
A summary of the MSP projects with engineering content, along with the project title, award number, and principal investigator is provided in Table 1 . The projects are presented in chronological order with the first two digits of the award number indicating the fiscal year of submission, which is usually also the fiscal year of the award. Note that several early awardees received subsequent awards as well.
Project | Title | Institution of Higher Education | Grant No | Principal investigator (PI) |
---|---|---|---|---|
A | El Paso Math and Science Partnership | University of Texas at El Paso | 0227124 | Navarro |
B | Teachers and Scientists Collaborating | Duke University | 0227035 | Ybarra |
C | SUPER STEM Education | University of Maryland Baltimore County |
0227256
& 0514420 |
Spence |
D | Partnership for Student Success in Science (PS3) | San Jose State University |
0315041
& 0953069 |
McMullin |
E | Math Infusion into Science Project (MISP) | Hofstra University |
0314910
& 0927973 |
Burghardt |
F | A Greater Birmingham Partnership: Building Communities of Learners and Leaders in Middle School Mathematics |
University of Alabama Birmingham
& Birmingham Southern College |
0412373
& 0632522 |
Mayer |
G | Project Pathways: A Math and Science partnership for Arizona Targeted Project Track | Arizona State University in partnership with Intel & Maricopa Community College system | 0412537 | Carlson |
H | Drafting a Blueprint for Educating Tomorrow's Engineers Today | Springfield Technical Community College | 0831698 | McGinnis-Cavanaugh |
I | UTeachEngineering: Training Secondary Teachers to Deliver Design-Based Engineering Instruction/ | University of Texas-at Austin | 0831811 | Allen |
J | Partnership to Improve Student Achievement in Physical Sciences: Integrating STEM Approaches (PISA2) | Stevens Institute of Technology | 0962772 | Sheppard |
K | Science Learning through Engineering Design (SLED) Targeted Partnership | Purdue University | 0962840 | Bowman |
L | LEADERS: Leadership for Educators: Academy for Driving Economic Revitalization in Science | University of Toledo | 0927996 | Czajkowski |
M | HR-PAL: Hampton Roads Partnership for Algebra | Hampton University | 1050389 | Akyurtlu |
N | CEEMS: The Cincinnati Engineering Enhanced Mathematics and Science Program | University of Cincinnati | 1102990 | Kukreti |
O | NUTURES: Networking Urban Resources with Teachers and University to Enrich Early Childhood Science | University of Toledo | 1102808 | Czerniak |
P | The NanoBio Science Partnership for Alabama Black Belt Region | Tuskegee University | 1102997 | Jeelani |
Q | The University at Buffalo/Buffalo Public Schools (UB/BPS) Interdisciplinary Science and Engineering Partnership | SUNY at Buffalo | 1102998 | Gardella |
Figure 1 presents the time frame of the projects. This emphasizes that although NSF's MSP program began in 2002, there is a marked and promising increase in engineering-related projects in recent years. This also means there is limited experience to draw upon to evaluate long-term impact.
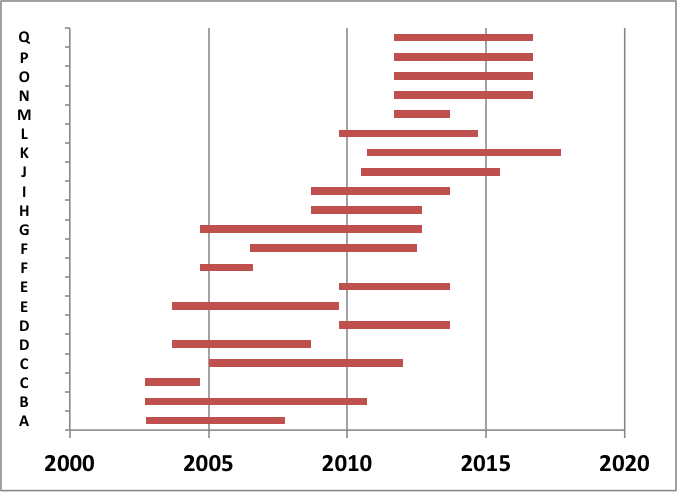
Engineering faculty involvement is summarized in Table 2. Engineering faculty provided professional development to K-12 faculty and helped develop engineering activities and curricular materials involving engineering design. Some engineering faculty members were tapped to serve as mentors. Engineering faculty members frequently serve as PIs, Co-PIs, and senior personnel on MSP projects. When their responsibilities are described, they tend to serve as consultants or mentors in developing engineering activities and curricula, as well as helping teachers to implement the activities in their classrooms.
Projects | Higher Education Engineering Faculty Involvement |
---|---|
C, E, I, J, K, N, O | Help design or develop professional development for inservice teachers |
E, H, I, L, N | Help deliver professional development for in-service teachers (typically in summer) |
D, H, I, L, P | Principal investigator on MSP grant is engineering faculty member |
L, P, Q | Co-PI(s) on MSP grant is engineering faculty member |
D, L, Q | Senior personnel on MSP grant includes engineering faculty member(s) |
B, N, O | Mentor in-service teachers during professional development |
G, K, P | Help develop K-12 curriculum |
D | Spend time in K-12 schools working on MSP project |
A, K | Receive professional development on how to work with K- 12 teachers |
A, N | Mentor undergraduate engineering students and STEM majors changing careers, working toward alternative teacher certification |
A summary of engineering content and the grade level impacted is given in Table 3. The dynamic of evolving science and math standards ensures that more resources will be directed to these efforts. For example, the recently revised Ohio State Science Standards are centered on real-world applications and connections to engineering. These projects suggest that design approaches and engineering solutions may be an effective way to connect science and math to students' daily lives. We note that the motivation for engineering in K-12 was presented in many proposals as a need for more engineers, a general need for a more scientifically and technically literate public, or both.
Project | Grade Level | Engineering Content |
---|---|---|
A | High School | Junior or senior level course which is inquiry based, addresses engineering concepts, and meets state mathematics and science standards. |
B | K-8 | Applications of electrical engineering such as solar power in inquiry-based science curricula. Contributions to "Teach Engineering: K-12 Resources" website: http://www.teachengineering.org/ |
C | Pre K-12 | Engineering emphasized through inquiry and problem/issue-based approaches. Industry engineers help develop & teach STEM modules - automotive, airplane & rocket engines; effects of stress on bridges & skyscrapers; factors involved in constructing roads & bridges; telecommunications. Mentor students, provide internships. |
D | K-8 | No apparent curricular development. |
E | Middle School | 26 math infused 8th-grade science units available on the MiSP website - http://hofstra.edu/misp addressing technological literacy standards. |
F | Middle School w/ Secondary Focus on High School | Examples include an engineering project on wound healing. http://www.eng.uab.edu/bme/labs/mathgrant/ |
G | High School | Teams of engineers, mathematicians and scientists partner with master teachers and STEM education faculty to generate portable instruction sequences incorporating engineering design. |
H | Middle School | Educational website about engineering for middle-school students ( http://www.talk2mebook.com ), primary goal is to motivate interest in engineering. |
I | High School | Designed a one-year high school engineering course in anticipation of Texas state standards for a 4th year engineering science course. Created learning outcomes - the narrative of engineering, engineering design skills, engineering habits of mind, design challenge topics. Units are energy generation; design, redesign and reverse engineering; and an extended design challenge. |
J | 3-8 | New curriculum focuses on energy concepts, is interdisciplinary and involves engineering. |
K | 3-6 | Science inquiry guided by an engineering design approach. |
L | K-12 | Engineering content on renewable energy and its environmental impact through Project-Based Science (PBS). |
M | Middle and High School | Engineering applications and practical examples for algebra, particularly in the area of robotics. |
N | Middle and High School | Engineering and science content employing design and challenge-based approaches in response to recently revised Ohio State Science Standards. |
O | Pre K- 3 | Science curriculum employing inquiry-based learning in formal and informal educational settings with input from engineering educators |
P | Middle School | Inquiry-based approach focused on NanoBio material science and engineering and 3-D simulations of concepts from their science curricula. |
Q | Middle School | Interdisciplinary, inquiry-based science and engineering design, such as tissue engineering and medical physics aligned with state science learning standards. |
A summary of undergraduate and preservice teacher involvement and opportunities is provided in Table 4. Major themes include recruitment of engineering students, creation of educational pathways for engineering majors to enter the teaching profession, and inclusion of engineering content and design in teacher preparation curriculum. One recurring recruitment strategy was for engineering students to work with teachers in order to enrich teacher engineering content knowledge.
Projects | Undergraduate and Preservice Teacher Involvement |
---|---|
F, H, K, D, C | New preservice coursework and degrees to better prepare teachers |
B, F, C | Internships in schools for preservice teachers |
E, P | MSP project assists with recruiting to existing preservice teacher programs |
A, L, Q | Undergraduate engineering students mentor or team with inservice teachers |
I, J, N, A | Teaching licensure pathways and recruiting for engineering and STEM majors |
M,O | (No apparent involvement of engineering undergraduates or preservice teachers) |
The role of in-service teachers was also evaluated. All but one project included summer and academic year professional development for in-service teachers focused on development of effective teaching practices and enhanced content knowledge (A-L, N-Q). Special foci included continuing education credits or advanced degrees (B, I, J, L, N), professional development for administrators in order to generate support and better understanding of issues (C, E, F, L), integration of technology into the classroom (E), creation of bilingual materials (G), and effective use of informal learning environments such as zoos, museums, etc. (O, Q).
Opportunities
NSF MSP funding has supported the creation of new initiatives to advance engineering education and models for collaboration. Examples include a new interdisciplinary Center for Engineering Education (I) and The Center for Technological Literacy (E). Professional development including teachers and engineering faculty has enhanced engineering faculty pedagogical skills (e.g., F). Industry engineers mentor high school students in a Future Teachers Club (C). One project was recognized by Microsoft Research University Program as a national K-12 outreach model (B).
Challenges
Early projects included in their reports to program officers some of the challenges to bringing engineering to K-12. Faculty time and responsibilities (teachers and professors) limited engagement with many aspects of the projects. Sometimes different engineering team members disagreed on how to adapt engineering to K-12. If the focus is interdisciplinary STEM or sustainability, science (or math) education focus can begin to dominate over time. Similarly, high stakes testing creates a drill situation, where engineering values of design and creativity are not included in measures of a school's success. To many, opportunities for creative thought are a benefit of engineering in K-12, but testing pressures may create practical implications for sustaining engineering efforts. As in many MSP projects, teacher content background and experience level vary widely. Selection of leadership team members must be done carefully and thoughtfully. The principal's support and leadership is crucial to sustaining teacher participation.
Conclusion and Future Work
This analysis gives an overview of efforts to implement engineering in K-12 through NSF's MSP program. These projects are employing many of the best practices in teacher preparation, professional development, curriculum development, and partnerships that characterize NSF's MSP program in general. Many programs had a focus on alignment of instruction and assessment of mathematics and science to meet state and national standards. Some programs had a focus on teacher preparation to meet the gap in prepared teachers, with alternate certification of engineering professionals or recruitment of undergraduate engineering majors. Some inculcated engineering content into preservice teacher education. Some projects provided support to minimize high turnover of new teachers. Industrial partners provided support to develop curricular materials or to serve as mentors.
We were surprised that so few projects created or strengthened teaching certification opportunities for engineering undergraduates. We view this as a promising practice for building capacity to support engineering in K-12. Despite arguments that engineering graduates can make much more money than teachers, demand could be surprisingly high due to the job security, geographic flexibility, and benefits afforded teachers. Similarly, we were surprised by how few projects had explicit goals to develop, archive, and distribute engineering curricula for K-12. There are notable exceptions: UTeach Engineering at University of Texas-Austin that is focusing on high school curricula, "Teach Engineering: K-12 Resources" that Duke University helped to launch, and SLED at Purdue University that is focusing on elementary curricula.
Many questions remain: Do we need separate engineering courses in K-12 or should it be embedded? If embedded, how should it be integrated? What is the required core of knowledge and how do we prepare teachers? How do we both prepare future engineering students and provide general engineering literacy? How do we promote diversity while incorporating engineering content? How will efforts be scaled-up? How will efforts be sustained?
Acknowledgements
We gratefully acknowledge the assistance of NSF MSP program officers James Hamos, Kathleen Bergin, Elizabeth VanderPutten, and Louis Everett in preparing this paper through reviewing portfolios and answering questions. We especially acknowledge the guidance of James Hamos in focusing this article. Any opinions, findings, conclusions or recommendations are those of the authors and do not necessarily reflect the views and policies of the National Science Foundation. Pamela Brown and Maura Borrego gratefully acknowledge that this material was based on work supported by the National Science Foundation, while working at the Foundation.
Pamela Brown ( PBrown@citytech.cuny.edu ) is Associate Provost at New York City College of Technology of the City University of New York. Maura Borrego ( mborrego@vt.edu ) is Associate Professor of Engineering Education at Virginia Tech. Both served as Program Officers in the National Science Foundation Division of Undergraduate Education between 2011-2012.
References
Baker, D. (2005). An intervention on tinkering and technical self-confidence, and the understandings of social relevance of science and technology. Paper presented at the 2004 National Association of Research in Science Teaching Annual Conference, Vancouver, Canada.
Beggs, J. M., Bantham, J. H., & Taylor, S. (2008). Distinguishing the factors influencing college students' choice of a major. College Student Journal, 42 , 381-394.
Custer, R.L. & Daugherty, J.L. (2011) Teaching engineering concepts in high school project. (2011). Journal of Technology Education. 23 (1) , 2-4
Fantz, T.D. & Katsioloudis, P.J. (2011) Analysis of engineering content within technology education programs. Journal of Technology Education. 23 (1) , 19-31.
Hall, C., Dickerson, J., Batts, D., Kauffmann, P. & Bosse. M. (2011). Are We Missing Opportunities to Encourage Interest in STEM Fields. Journal of Technology Education. 23 (1) , 32-46
Kuechler, W. L., McLeod, A., & Simkin, M. G. (2009). Why don't more students major in IS? Decision Sciences Journal of Innovative Education, 7 , 463-488.
National Academy of Engineering. (2009). Exploring Content Standards for K-12 Engineering Education (Project Summary). Retrieved from http://www.nae.edu/cms/14727.aspx , (accessed December 31, 2012)
National Research Council (2011). A Framework for K-12 Science Education: Practices, Crosscutting Concepts and Core Ideas. Washington, DC: National Academies Press.
National Science Foundation. (2008). National Impact Report: Math and Science Partnership Program. Retrieved from http://www.nsf.gov/news/newsmedia/msp_impact/msp_impact_report4_08.pdf (accessed June 22, 2012)
National Science Foundation. (2012). Math and Science Partnership (MSP). Retrieved from http://www.nsf.gov/funding/pgm_summ.jsp?pims_id=5756 (accessed June 22, 2012)
Ohland, W. W., Sheppard, S. D., Lichtenstein, G., Eris, O., Chachra, D., & Layton, R. (2008). Persistence, engagement, and migration in engineering programs. Journal of Engineering Education, 97 , 259-278.
Project Kaleidoscope (2006). Learning Science Volume IV: What Works, What Matters, What Lasts. Retrieved from http://www.pkal.org/documents/VIstudentLearningGoals.cfm%20 (accessed June 22, 2012
Silk, E., Schunn, C., & Strand C.M. (2009). The Impact of an Engineering Design Curriculum on Science Reasoning in an Urban Setting. Journal of Science Education and Technology, 18 (3), 209-223. doi: 10.1007/s10956-009-9144-8