JOTS v26n1 - Industrial Research and Development Labs and How They Inform Science and Technology Curricula
Industrial Research and Development Labs:
How They Inform Science and Technology CurriculaMarc J. de Vries
Science and Technology Education
One of the struggles in the development of technology education is the establishment of a proper relationship between science education and technology education ( de Vries, 1997 ). In part this is because science educators hoped to integrate technology into the school curriculum to eliminate the need for a separate area for teaching and learning about technology. This model has yielded programs often based on the "technology is applied science" paradigm. Even though this paradigm has been challenged heavily by historians and philosophers, we still encounter this model.
By contrast, since science education already is a well-established school subject, no one debates whether science should be a separate area of teaching and learning in the curriculum. But the place of technology education still requires some defense. This defense is sometimes sought by identifying a body of knowledge, a discipline, expressly for technology (either a combination of engineering disciplines or something entirely new). Some authors challenge this defense, but there is certainly evidence there are categories of knowledge in technology which differ from science ( de Vries & Tamir, 1997 ).
This still leaves us with the question: What then is the proper relationship between science and technology education, assuming both have the right to serve as separate areas of teaching and learning in the curriculum? One way is to look at how science and technology interact in reality. In fact, we would have to find a place where people consciously try to make science and technology interact optimally. But where do we find such situations?
Industrial research laboratories certainly belong to this category of places. In an industrial research lab, scientific research is performed, but for the sake of technological developments. The people working there find themselves in a sort of double role: On the one hand, they want to be serious scientists, who publish in well-respected scientific journals; on the other hand, they are well aware of their position within the totality of the industrial company, where new products and processes must be developed in order to survive financially. Studying the way these people have historically struggled to find a proper position within the company offers a way of understanding these science-technology interactions, some of which have been more successful than others. Naturally, the extent of their success must always be judged against the background of the socioeconomic circumstances of that time.
The next question is: Is there any material available for this sort of study? The answer is: Yes, some material exists and more is being developed. Some of the major industrial research laboratories, such as the famous Bell Labs, General Electric Labs, or the Du Pont Labs-all in the United States-already have their written histories published. Some major industrial research labs are having their history written right now, for example, the Philips Research Labs. For some major industrial labs, such as the Siemans Laboratories, no written history yet exists. Some smaller labs have a written history, too. In this article I mainly draw from the historiographies of larger research labs, since they yield the richest material for the type of analysis I wish to make.
Patterns of Interactions Between Science and Technolog
What types of science-technology interactions do we find in the histories of the various industrial research laboratories? In this section I explore three interactions (in chronological order).
Science-and-Technology
The first type of interaction is the almost total integration of the industrial research lab in the overall company. One might describe this complete immersion using the term science-and-technology, as if it were only a single concept rather than two separated by the word and. That is the sort of interaction found in the early history of the companies, when they were still small and their organization was often very informal. In fact, the rise of independent laboratories in such companies is one of the aspects that Chandler ( 1977 ) called "the visible hand" in industrial organizations: The management of those companies created a strategic model with distinct functions, such as R&D, production, marketing, and sales. The contacts between people working in the research lab and people working in the rest of the company were based primarily on personal acquaintances. Sometimes these contacts were so direct that it was difficult afterwards to identify exactly where the boundary existed between research activities and product and/or technology development. At the AT&T Labs, it is well known that between WWI and WWII, entire labs were involved in almost all innovation phases ( Reich, 1977 ).
In the case of the Philips Research Labs, we also find similar interactions during this period. Work in the area of X-ray tubes was initiated because of the personal contact between Anton Philips, one of the owners of the company, and Gilles Holst, who was in charge of the lab during that period. Philips was concerned about health care in the Netherlands and the problems of hospitals obtaining adequate X-ray equipment when there was no more deliveries from Germany because of WWI. At first, Holst had some of his researchers repair X-ray equipment from hospitals and simultaneously do research on that equipment. When they started yielding improved and new types of X-ray tubes, such as the Metalix and the Rotalix tubes, a pilot production was started. In the end this resulted in a new business area for the company that still exists today.
In the same way, various new areas emerged and the product range diversified substantially in the years between the two world wars; the research labs continued to function as engines for new options for the factories. This is not to say that the lab always took the first initiative, but the lab certainly played an important role in enabling the company to diversify into new areas. In general, topics in the research program were almost always directly related to products which interested the whole company and some were already in production. Thus, a lot of new topics emerged out of research that was related to light bulbs, originally Philips' sole product ( Blanken, 1992 ). For example, when research was done on the negative phenomena of gas discharges in light bulbs, the research lab in the end came up with new types of lighting devices based on this phenomenon (gas discharge lamps).
In the GE Labs, a similar development took place that was regarded as a model to be followed by several Philips researchers. In that respect, Wise ( 1985 ) mentioned the contacts between GE Labs and Philips scientist Balthasar van der Pol. The extent to which this way of working was good for the company must be judged against the circumstances that were then favorable for such a development. But later, when Philips had to be more selective in the fields in which it wanted to operate, the almost endless diversification became a problem rather than a stimulus. Relying on scientific developments did not yield a good mechanism for selecting areas that were to be counted as "core business." Here other sorts of considerations have to be made, such as market analyses that certainly did not belong to the competencies of the research labs. The GE Labs had a similar function for its "mother" company ( Reich, 1985 ). Here, too, the lab played an important role in the product diversification of the company.
In summary: A situation in which the activities of the research lab are almost indistinguishable from the commercial activities of the factories (and later on from the product divisions) allowed the company to enter new areas of products and technologies. This, however, did not yield an efficient mechanism for selecting those areas in which the company wanted to really become competitive.
The Industrial Research Lab as an Ivory Tower
Perhaps this is the popular image of any industrial research lab: a place where scientists of a high level are allowed to do scientific research based entirely on their own interests without any conscious concern for what the rest of the company might ask for. This image is fed by stories of inventive individuals who have come up with wonderful ideas for new products and technologies without a request from any business group or product division. It is the genius of these individuals that results in good ideas and not a specific "market pull"- as we would call it today.
Industrial research labs did function this way, particularly in the 1950s and 1960s. It was stimulated by a strong belief in the importance of fundamental scientific research. This belief was expressed in a well-known report by President Roosevelt's scientific advisor, Vannevar Bush, entitled "Science, the Endless Frontier." Bush showed that WWII had yielded many opportunities for new technologies by developing and exploiting new knowledge of the fundamentals of nature. Here we have to be a bit careful with the use of the word fundamental in this discussion. Fundamental often means a concern with the basic structures of matter, but here the same term concerns the phenomena underlying the functioning of products. For Bush, the first type of fundamental research was very important. His report was one of the reasons several industrial research labs enhanced their fundamental research capacity. This was an important stimulus for the emergence of science-based industrial developments ( Noble, 1979 ).
In the Bell Labs, for example, it stimulated the study of solid-state physics. This most certainly was one of the main reasons the transistor was invented by Bell Labs. First efforts to design this new device on the basis of trial and error (and the use of analogies with triodes) did not result in a well-understood and reproducible device, even though it did yield the so-called point-contact transistor that could amplify an electrical current. However, the real breakthrough did not come until knowledge of solid-state physics was applied to this problem ( Sarlemijn, 1993 ). This resulted in a device that has certainly become one of the most important innovations in the 20th century.
Equivalent evidences for the success for such an approach can be found in the history of the Philips Research Laboratory. After WWI, the Philips Research Labs found itself to be an autonomous lab in the midst of autonomous product divisions ( Blanken, 1998 ). That caused a variety of transfer problems ( de Vries, 1999 ), as we will also see later. Favorable economic circumstances allowed for a great deal of free research, whereby the lab did not suffer from the problems of its main European competitor, the Siemens Labs, where researchers' work in certain areas was forbidden by the Americans ( Pfisterer, 1987 ; Trendelenburg, 1975 ). It also allowed for a great deal of new research in which quantum mechanics played an important role. In that respect, it was quite suitable that the research representative in the Philips Board of Management, Dr. Hendrik Casimir, was a well-respected physicist who himself had contributed to the development of this new scientific theory ( Casimir, 1983 ).
In the case of the Philips Research Labs we can point to the invention of the so-called Plumbicon, a television pickup tube that is still used in professional cameras. It was developed mainly due to the use of solid-state physics as a selection mechanism for optional target materials that would have the necessary photoconductive properties ( Sarlemijn & de Vries,1992 ). But the same example shows the problems of such an approach. The research lab in this case had great difficulties in transferring the device to the product division. Even though the lab had succeeded in making prototypes of the pickup tube, the production of larger quantities appeared to be quite problematic. Mass production is not really an appropriate term here, given the limited numbers of pickup tubes that were needed to supply the broadcasting companies. And here it was not so much fundamental research that helped to solve the problem, but a good engineer's fingerspitzengefühl (intuitive knowledge by experience). Although at first glance the Plumbicon seems to be an example of applied science, the same case study undermines this paradigm as we look further into its development.
When we look into the history of the Philips Research Labs, and we count the number of successful innovations that emerged in this way from the research labs in the 1950s and 1960s, we only find two important innovations: the Plumbicon and LOCOS. LOCOS stands for LOCal Oxidation of Silicon. This technology is used to produce integrated circuits, which for a long time was the dominant technology for all IC producing companies. Thus it yielded enormous license incomes to Philips and ranks as one of the greatest successes of the company. These two major innovations in a 20-year period counterbalanced a lot of research outcomes that never became successful.
The research program was very expansive in that period. It became evident that this way of working was no longer suitable when the amount of effort spent on research is reduced while the rate of successes remains the same. Only one or perhaps even no big success at all came out of all of this. Of course, such a result can not be afforded by a company for very long.
There are case studies that show how costly nonhits can be using this ivory tower strategy. In the first place, we can point to the video disc. Both Philips and RCA Labs worked on this optical recording system. In the RCA Labs it was seen as an important project through which the lab could regain its status as an innovation center for the company following a difficult period of personnel reduction after the lab had made a wrong strategic decision in computer research ( Graham, 1986 ). Like the Philips Research Labs, the RCA Labs functioned as an autonomous lab in the midst of a number of autonomous product divisions. A choice was made to elaborate the optical storage technology, even though some other options had been tried and not yet completely rejected (e.g., Photopix with small pictures on a disc and Discpix with capacitive storage of video information).
A large market was expected even though there was no hard evidence for that. And when-after many years of intensive research-a working product finally could be delivered, the market that the lab had expected appeared to be nonexistent; only small numbers of video discs were sold. The Philips Research Labs went through a similar process, but they could afterwards claim that the same optical recording knowledge that had been gained in the video disc project later on could be used to develop the compact disc, unquestionably a successful product, in a relatively short time. However, the development of the compact disc was not an example of an ivory tower process, but of the sort of process that will be described earlier.
For the Philips Research Labs there is another famous example of ivory tower research with failing commercial results: the hot air engine, or Stirling engine ( de Vries, 1993 ; more detailed information can be found in Gradstein & Casimir, 1966 ). Due to the high degree of freedom for the researchers, this project was continued for many years even though not a single product division showed any interest in the engine. Finally, in 1979, the project was stopped after more than 40 years of research. It is evident that this sort of failure is inevitably a risky aspect of an ivory tower industrial research situation.
Customer-Oriented Science
In the 1970s most industrial companies had to redirect their research programs because of changing socioeconomic circumstances. In the first place, a lot of criticism against the place of technology in society emerged. At the same time, economic growth diminished and customers became more selective. It was no longer true that any new product would naturally find a substantial group of buyers. Industrial companies had to be more selective in putting new products into the market. Thus a company's business or product division influenced the research programs.
This process can be seen not only in the history of the Philips Research Labs, but also in the history of the Bell Labs, the General Electric Labs, and the Du Pont Labs. In fact, Philips was one of the slowest to make such a transition because it kept its research program structured according to disciplines rather than products (the three main groups in the Philips Research program remained: materials (mainly with chemists), devices (mainly with physicists), and systems (mainly with electrical engineers). Most other labs changed the structure of the research program to enable a better match with the product-oriented structure of the rest of the company (for Du Pont, see Hounshell & Smith, 1988 ).
For most research labs, this change soon meant that they had to approach product divisions within their company to get contracts for them. This was another process that was postponed until the 1990s in the Philips Research Labs, but in the end was forced upon them too by the company's Board of Management ( van Gruijthuijsen & Junge, 1992 ). One of the major struggles in this process was to find a balance between freedom for the lab, which would enable researchers to keep doing long-term research, and mutual commitment between the research lab and the product division. Lack of this commitment, in the case of the Philips Research Labs, had frustrated a fruitful customer orientation (the customer being the product divisions of the company) in the 1970s and 1980s. Even though a lot of formal contacts were established between the research labs and the product divisions, often the cooperation did not end with a useful product, but instead could be easily broken off by either of the two parties. It was only the introduction of contract research and a new way of financing the research program (no longer by a lump sum directly from the Board of Management, but to a large extent from the budgets of the product divisions for targeted research, through which the product division was to define the targets) that brought a solution to this serious problem.
When we look at the outcomes of these changes, we see that the research labs no longer yield many dramatic breakthrough innovations, but numerous smaller contributions to product developments. The lab serves as a continuous source of know-how in a variety of quite different areas. One of the major successes of the Philips company that resulted from this type of science-technology interaction is the compact disc. Contrary to the Plumbicon, this idea did not come from the research lab but from one of the product divisions. But its development required know-how from very different areas, such as optical recording, signal processing, and IC design and technology. All these know-how areas were present in the lab; thus a combination of these different areas could be used to achieve the desired product ( Ketteringham & Nayak, 1986 ; Lang, 1996 ). In fact, a lot of this expertise was gained in the development of a product that is still an example of the previous way of working (in which the lab served as an ivory tower), namely, the video disc (for example, the RCA video disc as an example of ivory tower behavior). Commercially the video disc never became successful, but it resulted in the knowledge that later would be used to support the development of the compact disc (certainly a major success for Philips).
Science in Technology Education
We have seen three types of science-technology interaction by studying the history of industrial research laboratories:
- A very direct relationship, whereby the two are almost integrated and science serves as a pushing force.
- A situation in which the two are quite distinct and technology is the result of selecting and elaborating ideas that emerge from scientific research.
- A situation in which scientific research is done on demand by technological developments.
All of these approaches are successful in a way, andeach has also caused problems. The science-and-technology situation enabled smooth transfer of ideas but did not provide good selection mechanisms for technological developments. Fortunately in the years when most industrial labs worked according to this type of interaction, this was not a problem because of the social and economic circumstances. The ivory tower strategy yielded a number of real breakthrough successes but was often accompanied by difficult transfer processes. Also, this was not really a problem because the company could afford a great number of non-hits.The customer- oriented research strategy resulted in a constant flow of knowledge that enabled incremental, rather than breakthrough, product developments.
In a way these categories seem to be not so far away from Layton's cathedral, quarry, and store roles of science education ( Layton, 1993 ), which were derived from a methodological rather than a historical approach. But some differences exist. The role of science as "cathedral" for technology, in Layton's terms, means that fundamental science is performed and technology can study it to learn research methods. "Quarry" means that technologists take from science what they think is useful and leave the rest alone. Finally, "company store" is meant to explain the way that scientists take into account the needs of technologists when developing and presenting their studies. What we have seen in the history of the industrial research labs is a cathedral and quarry role in Section 2.2 and a company store in 2.3. What seems to be missing in Layton's analogy is a role as described in 2.1, whereby the work of scientists and the work of technologists are so closely related that they almost seem to be integrated.
What can we learn about the way scientific knowledge and science education can interact with technology education (because that was the original question that triggered us to make our historical comparisons)? Primarily it is important that pupils become aware of the different roles science can play in technology. Often technology education curricula do not seem to differentiate clearly enough between different types of technology. The focus is often on what is common in all technological developments. This serves as an effective basis, but we should make clear that all the commonalities do diminish the notion that one technology is not necessarily the same as another. One of the aspects that differentiates them is the role of science.
One way of considering this differentiation in technology education is by doing what we did in this article: reviewing the way different products were developed in different periods of the history of industrial research laboratories. Historical insights should be part of technology education anyway; we can make them fruitful by using them to illustrate the difference between various types of science-technology interactions.
But there is more. As we have seen, the different science-technology relationships all have their strengths and weaknesses. In technology education we should build relationships with science education in such a way that we enhance the strengths and avoid the weaknesses. Let us look again at the three interaction patterns we have seen.
A "Science-and-Technology" Pattern in the Curriculum
The science-and-technology pattern would have as its educational equivalent the integration of science education and technology education. As in the industrial research laboratories, this will probably result in science being the main driving force behind technology. Those technologies will be dealt with in the curriculum where scientific research played an important role. Such an approach will result in a great variety of possible issues (as in the industrial company a variety of new industrial activities emerged out of this science-technology cooperation). But it may not provide a useful selection mechanism to help us find those issues that are really relevant for the "market," that is, our pupils. What do they really need in their future life in a high-tech world? Such information is not necessarily taught when science defines what is worth integrating in the curriculum and what can be left out. Thus we can conclude that this option can only be successful if we have many teaching hours available since the number of issues that must be dealt with is very large. There must be time to cover at least a number of topics that are relevant for the pupils from a social perspective. Just as we saw in the science-and-technology pattern, it did well in a time when many new products could be developed in an almost risk-free atmosphere because there would always be enough output to generate revenue.
An Ivory Tower Pattern in the Curriculum
The ivory tower pattern has its educational analogy in science education and technology education when they are separate and do not communicate with each other, but technology education selects certain issues from science education (a company store role of science education) and learns the lesson that concept teaching, which is the key issue in science education, is important (a cathedral role of science education). The history of industrial research labs suggests that this will result in breakthroughs for technology. That may hold when we make the comparison with education: It would be a major step forward if we were able to build up a technology education curriculum that-from a conceptual point of view-is as strong as the science education curriculum. Likewise, the understanding of technology would be greatly enriched if we would show pupils how sophisticated use is made of natural phenomena. But we should certainly be aware of the pitfalls of this pattern: The transfer from science to technology can be most problematic in this case. It requires a very good professional relationship between science teachers and technology teachers if this is to work out well.
A Customer-Oriented Science Pattern in the Curriculum
Finally, we have the option of the customer-oriented science pattern. Here science will adapt to the needs of technology. A skeptic might say that a miracle has to occur if this is to become practice in schools. In the history of industrial research labs, we have seen that it only became a reality because it was forced upon the labs by the higher management of their respective companies. The same might be true in education: We can hardly expect that this well-respected and established school subject will adapt to the "newcomer," technology education. Yet, the history of industrial research labs shows us that, particularly when time and resources become scarce, this interaction pattern could be quite fruitful. Of course some opportunities for science/science education for its own sake should remain, but a substantial part could serve effectively as an input for technology/technology education when it is developed and presented in such a way that it can easily be absorbed by technology. The history of industrial research labs shows us that two elements are needed for this to occur:
- Mutual commitment: Science education should commit itself to provide relevant input for technology education and technology education should commit itself to make use of this input.
- Shared project work: Science education and technology education should work together in order to understand each other and to be effective in their relationship.
When these two conditions are fulfilled, the customer-oriented science pattern will prove to be as fruitful as it did in the industrial labs situation. It may require a lot of effort before we get there, but as long as time and resources are limited it is worth pursuing this approach. In the end both science education and technology education would benefit from these collaborations and commitments.
Marc J. de Vries is a professor at the University of Technology in the Netherlands. He is a member of Eta Field chapter of Epsilon Pi Tau and received his Laureate citation in l998.References
Blanken , I. J. (1992). Geschiedenis van de Philips Electronics B.V. (History of the Philips Electronics, Inc.; in Dutch), Vol. 3. Leiden: Martinus Nijhoff.
Blanken , I. J. (1997). Geschiedenis van de Philips Electronics B.V. (History of the Philips Electronics, Inc.; in Dutch), Vol. 4. Zaltbommel: Europese Bibliotheek.
Casimir , H. B. G. (1983). Haphazard reality: Half a century of science. New York: Harper & Row.
Chandler , A. D. (1977). The visible hand. S.l.: Belknap Press.
Gradstein , S., & Casimir, H. B. G. (1966). An anthology of Philips research. Eindhoven: Philips Gloeilampenfabriek.
Graham , M. (1986). The business of research: RCA and the video disc. Cambridge: Cambridge University Press.
Gruijthuijsen , E. van, & Junge, P. (1992). De revolutie van Jan Timmer (The revolution of Jan Timmer; in Dutch). Naarden: Strengholt.
Hounshell , D. A., & Smith, J. K. (1988). Science and corporate strategy: Du Pont R&D 1902-1980. Cambridge: Cambridge University Press.
Ketteringham , J. M., & Nayak, P. R. (1986). Breakthroughs! New York: Rawson .
Lang , J. (1996). Das compact disc digital audio system: Ein Beispiel für die Entwicklung hochtechnologischer Konsumelektronik (The compact disc digital audio system: An example of a high tech consumer electronics development; in German). Aachen: Technische Hochschule.
Layton , D. (1993). Technology's challenge to science education: Cathedral, quarry or company store? Buckingham: Open University Press.
Noble , D. F. (1979). America by design: Science, technology and the rise of corporate capitalism. Oxford: Oxford University Press.
Pfisterer , H. (1987). Siemens research laboratories. Phys. Technol., 18, 32-40.
Reich , L. S. (1977). Radio electronics and the development of industrial research in the Bell system. Baltimore: John Hopkins Press.
Reich , L. S. (1985). Science and business at GE and Bell, 1876-1926. Cambridge: Cambridge University Press.
Sarlemijn , A. (1993). Designs are cultural alloys. In M.J. de Vries, N. Cross, & D. P Grant (Eds.), Design methodology and relationships with science (pp. #) Dordrecht: Kluwer Academic Publishers.
Sarlemijn , A., & Vries, M. J. de (1992). The piecemeal rationality of application oriented research: An analysis of the R&D history lead ing to the invention of the Philips Plumbicon in the Philips Research Laboratories. In M. Bakker & P.A. Kroes (Eds.), Technological development and science in the industrial age. Dordrecht: Kluwer Academic Publishers.
Trendelenburg , F. (1975). Aus der geschichte der forschung im Hause Siemens (From the history of research in the Siemens Company; in German). Düsseldorf: VDI Verlag.
Vries , M. J. de. (1993). The Philips Stirling engine: A historical-methodological case study into design process dynamics. Methodology and Science, 26, 74(86.
Vries , M. J. de. (1997). Science and technology teacher training: What kind of training for what type of teaching. European Journal of Education, 32(1), 59-73.
Vries , M. J. de. (1999). Technology transfer as a major concern of the Philips Research Laboratory Management. In M. Inzelt & J. Hilton(Eds.), Technology transfer: From invention to innovation. Dordrecht: Kluwer Academic Publishers.
Vries, M. J. de. & Tamir, A. (1997). Shaping concepts of technology: What concepts and how to shape them. In M. J. de Vries & A. Tamir(Eds.), Shaping concepts of technology: From philosophical perspectives to mental images. Dordrecht: Kluwer Academic Publishers.
Wise , G. (1985). Willis R. Witney, General Electric and the origin of US industrial research. New York: Columbia University Press.
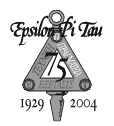

TS