JOTS v34n1 - STEM-Based Computational Modeling for Technology Education
STEM-Based Computational Modeling for Technology Education
Aaron C. Clark and Jeremy V. Ernst
Abstract
According to professionals in education, change is an ever-present and evolving process.With transformation in education at both state and national levels, technology education must determine a position in this climate of change.This paper reflects the views on the future of technology education based on an ongoing research project. The purpose of the project is to show a contemporary view of one direction that technology education can take for providing 21st century skills and learning to students.
Change in Technology Education
Over the years, the technology education profession has experienced several changes in the content taught and the way the field is presented. From the manual arts movement to the Jackson Mills Project, change and the ability to refocus curricula has been central to the identity of the technology education profession. One of the reasons students and society benefit from modernized technology education is because of its willingness and ability to anticipate and identify necessary change ( Gomez, 2001 ). Computational modeling is one example of contemporary technology that can be taught in the classroom and allow students to acquire 21st century skills. This capability to identify areas of change, allows the profession to grow and take on new endeavors that have resulted in the discipline remaining contemporary ( Partnership for 21st Century Skills, 2004 ).
The current trends in education that are related to the discipline include areas of integration, academic accountability, and a variety of literacies ( Partnership for 21st Century Skills, 2004 ). Although educators in the field have embraced a need for technological literacy, associated standards, and the integration of content in the areas of science, technology, engineering, and mathematics (STEM), few have evaluated the role in the No Child Left Behind Act ( U.S. Department of Education, 2007a ), the President’s Information Technology Advisory Committee (PITAC) Report ( National Coordination Office for Networking and Information Technology Research and Development, 2007 ), or the new Perkins Act ( U.S. Department of Education, 2007 ), and what impact the initiatives have on the future. The intent of this paper is to define the authors’ views on the direction of technology education for the next 10 years.
Current Changes in Technology Education Curriculum
Looking into skills for the 21st century, authors Murnane and Levy (2004) stated that for the United States to remain globally competitive, new skills such as expert thinking and complex communication need to appear in curricula at all levels. Expert thinking addresses abilities, such as critical thinking skills and creativity, required to solve problems outside traditional frameworks. Complex communication addresses the need to have students breakdown information and communicate in different forms and in a variety of ways to a diverse set of audiences.
Considering the competencies outlined by Murnane and Levy (2004) , how can technology educators, during the next 10 years, bring expert thinking and communication skills into the classroom? The authors of this paper suggest focusing on a national technology education curriculum that can transform the discipline to include engineering, design, and computational science (i.e. computation modeling) as new areas of study underneath the broader umbrella of STEM education. Engineering, design, and computational science, through the study of technology, will permit the use of higher order thinking skills, the integration of academic areas, and the placement of a broader focus in areas needed for future economic growth in the United States.
Engineering education can be used to bring about career awareness for those students wish-ing to become professionals in engineering- and technology-related disciplines or as a way to link physical sciences to technology for real-world understanding (Varnado & Pendleton, 2004) . Modeling, testing, analysis, and simulation could all be major components of this type of technology education curriculum. The study of engineering, through a course for all students or a course for those wishing to pursue engineering as a career, helps address expert think-ing, established by Murnane and Levy (2004) .
Design concepts also can be easily integrated to address expert thinking and particularly, complex communication. Although design has been a part of the technology education curriculum since its beginning, only with the development of the Standards for Technological Literacy (STLs) has it come to the forefront. The authors suggest that during the next 10 years, technology educators should find a unique way to present design through the study of technology. One suggestion would be to not solely concentrate on traditional areas within design education, but to classify design into three categories that can be easily supported by the STLs. Design curricula could present information as related to three worlds: micro, human, and macro.
The micro world would use design to problem solve and reconstruct at the level that is normally invisible to the human eye. This would allow for the inclusion of scientific concepts (i.e., nanotechnology and areas of biotechnology and biometrics) involving data-driven simulations. The human-built world is where most professionals see design components being positioned. Design at this level includes a variety of areas of problem solving, including re-engineer-ing a variety of devices for improvement. The human-built world of design could include, but not be limited to, the traditional areas of graphic and industrial design. Finally, the macro world, within a 21st century design curriculum for technology education, would include the architectural, civil, and transportation areas. This would encompass the study of civil structures, environmental design, and community planning (International Technology Education Association, 2005) .
Changes to existing national curricula focused on technology education are currently being defined by the profession and may become a part of technology education within the next 10 years. But what can be done to address the two skills of expert thinking and complex communications as a collective unit? How can the professionals in the area of Technology Education address the current problems of high dropout rates, teaching 21st century skills to all students, and bringing relevance to the classroom? Additionally, what roles do expert thinking and complex communications play in technology education? The authors of this paper believe that the inclusion of computational science will assist in addressing issues related to drop-out rates and 21st century skills.
Computational science, as defined in this paper, comes from the extensive research con-ducted for the development of a new scope and sequence for technology education in North Carolina. Computational science within technology education will aid in the integration and enhancement of STEM-based education. The National Coordination Office for Information Technology Research and Development sponsored a presidential taskforce to look into 21st century skills. A product of this taskforce was the establishment of the President’s Information Technology Advisory Committee (PITAC). The PITAC (2005) defines computational science as the ability to arrive at solutions to real-world problems through computing applications. This definition further includes areas of modeling, simulation, computer science, information science, and computing infrastructure to support areas of science and engineering in solving problems. Members of the PITAC consider this area as a multidisciplinary approach to address-ing 21st century challenges, and thus view visualization as a key to complex communication across disciplines.
Considering this definition and all the components associated with the report, the authors of this paper determined that computational science would be the next area for study at both the state and national levels. Computational science at the secondary level includes the use of multi-disciplinary approaches to learning (i.e., STEM integration), tools (i.e., computers), and techniques (i.e., real-world scenarios) that can attract students, especially those deemed at risk for dropping out of school. At-risk students are defined, in this paper, as “students whose economic, physical, emotional, or academic needs go unmet or serve as barriers to talent recognition or development, thus putting those students in danger of underachieving or dropping out” (National Association of Gifted Children, 2008, ¶ 8) .Computational science will allow for the integration of science and technological literacy to occur though the study of visualization and the development of both virtual and physical models. This definition was developed so that true STEM integration could occur in the technology education classroom at the same time that 21st century skills for students taking technology education courses are being developed. The authors believe that this new area will be important as technology educators try to reach and support both state and federal initiatives while maintaining the intended focus: technological literacy for all.
STEM in the Curriculum
Hevesi (1999, 2007) reports on a research study conducted by the Comptrollers Office in the City of New York that identified three major skill and knowledge indicators of workforce success after high school: (1) mathematics competency, (2) science competency, and (3) technological competency. Hevesi indicated that students are poorly prepared, academically, in mathematics and science in early grades, hampering knowledge growth in advanced mathematics and science courses in later educational endeavors. Hevesi (2007) also indicated a teacher training shortage in mathematics and science disciplines. An evaluation of the findings from the study led to the recommendation of integrated content across science, technology, and mathematics with a supportive teacher professional development structure.
Considering this need to bring about STEM and the different competencies needed for the future workforce, the authors of this article began the process of developing a STEM model for technology education that would address Murnane and Levy’s (2004) two central skills, expert thinking and complex communication. The skills need to be addressed in such a way that supports initiatives important to the state and nation. This would include working with students deemed at risk for successful completion of end-of-grade tests for academic areas. Given that engineering and design are already established areas of study, researchers and educators in North Carolina wanted to see how computational science could be used to educate at-risk students, while bringing about technological literacy. Research has already been conducted on design and engineering education for secondary education, but none had been used to investigate computational science as defined in this paper. Therefore, the group set out to find a way to integrate STEM into the technology education classroom through the area of computational science.
The initial investigation included computational science fundamentals and relied on a companion course structure with a full theoretical foundation. This model became too complex, and teachers lost focus and were unable to achieve the collaboration necessary for the model to serve as an effective educational approach. The researchers decided that a supple-mental companion approach, as opposed to an integrated companion approach, would be easier to implement. Such a supplemental approach targets specific academic content, whereas the integrated approach spans multiple core areas simultaneously. The research described in the next section was conducted for the development of a STEM-based curriculum for technology educators in North Carolina; as it also illustrates the demonstration of power that computational science can have on the future of technology education worldwide.
The North Carolina STEM Project: A Future Model for Technology Education
The North Carolina STEM project (NC-STEM), sponsored by the North Carolina Department of Public Instruction Career and Technical Education division and North Carolina State University, was designed to aid in the endeavor to keep at-risk high school students in school. The project gave students additional help in the areas of mathematics and science (which required a passing grade for graduation) using career and technical education (CTE) con-tent and proven pedagogical methodologies such as kinesthetic learning applications and problem-based learning (Stone & Alfeld, 2004) . The NC STEM project evolved from research developed during the past decade that had influenced the development and funding of curriculum projects such as the Scientific and Technical Visualization curriculum and the National Science Foundation instructional materials development project titled, “VisTE: Visualization in Technology Education” (Clark, Wiebe, Petlick, & Ferzli, 2004) .
With North Carolina’s need to improve its drop-out rate, the integration model was applied to core academic areas using methods and con-tent from CTE for piloting and further develop-ing NC-STEM. Note that most models include the integration of academic areas (science, technology, and mathematics) focusing on higher cognitive understanding that lead toward the advanced understanding of engineering, science and related STEM careers (Brown, 2003) . This project was designed to use previously described integration fundamentals with those students who were at risk of dropping out of school, not with the academically gifted.
The researchers of this project believed by making required academic materials relevant to students deemed at risk of failing and dropping out of school, they were more likely to under-stand the content and pass the end-of-grade tests and therefore stay in school and graduate. Although myriad academic courses exist, the researchers felt that the first two courses that demanded this type of development and work were algebra and biology, since passing grades in both are required for graduation in North Carolina, as well as in other states (Reddick, Jacobson, Linse, & Yong, 2007) .
The project began in academic year 2005-2006 with the development of a theoretical framework that included the teaming of academic subject teachers with those in CTE (mainly teachers in technology and graphics education). Teachers were to work together to develop and test materials that both academic and technical teachers could use in the classroom to enhance fundamentals in biology and algebra. They were asked to focus on areas within the state curriculum where academic subject area teachers identified a lack of student understanding. Three pilot sites were selected within the state, all rep-resenting a population of at-risk students within their school deemed appropriate for this project. Due to lack of teacher understanding, misaligned pacing guides, and inadequate time to cover requirements in the academic course, this first try was a failure. Further investigation was predicated on the observations made from the prior project, where preliminary exploration within computational science took place.
STEM Companion Model
In the academic year of 2006-2007, the researchers decided not to continue with the above-mentioned model. Given their collective experiences and through a careful review of additional literature in the field, the researchers decided to develop a new theoretical model that would fully capitalize on Computational Science in an applied manner (Cushman, 1989) . It was taken into considering how the PITAC report could be applied in secondary education; this new model would focus on literacy within science, mathematics, and technology, as well as the visual and kinesthetic learning associated with CTE areas, especially technology education. This model was still focused on the two required courses of algebra and biology, and it also incorporated pedagogical methodologies brought forth by CTE. The model also required that a course be made for each academic subject in question (i.e., one for algebra and one for biology).
The companion courses were designed to use a “hands-on” approach to learning, having students use both virtual and physical modeling in the process. The researchers decided not to focus on the academic course competences, but instead focused on major topic areas in the “end-of-grade” exam that students have difficulty with as indicated by teachers and statewide statistical data (Public Schools of North Carolina, 2007) .
Considering this new model, the researchers met with teachers, administrators, and pilot test sites throughout the state to decide on an initial plan of action. From the meetings, a model was developed and piloted in two field sites. This new STEM companion model required at-risk students to not only take the required academic courses but also to take CTE-based companion courses at the same time to further develop their knowledge in that subject matter and focus on areas of difficulty. Students in the course who were deemed not at risk were not required to take the companion course.
The companion courses are not designed to replace the existing academic course, but to compliment the required knowledge and provide students with additional time, activities, and different methods of learning for obtaining the essential information. During this research, it was decided to focus only on one course. Algebra I was selected because it was identified as a major stumbling block for students statewide.
The researchers began the process by find-ing teachers in both the academic area of mathematics and in CTE areas of technology education and graphics to develop this new companion course for Algebra I (Public Schools of North Carolina, 2007) . Teachers were charged with the identification of problem areas for most students in Algebra I, and from this they developed virtual and physical modeling activities that could help students better understand the identified areas. Mathematics and CTE teachers identified the following areas as those with witch students need the most help:
- Rational numbers
- Irrational numbers
- Geometric patterns and equations
- Interpreting graphs and using formulas for interpretation
- Understanding ratios
- Slope
- Quadratic equations with outputs
- Understanding exponential functions
- Using formulas to solve problems pertaining to exponential functions and analysis
Activities to teach the identified topics included the use of computer-aided design, web-based gaming applications, and developing PowerPoint Presentations. Instructional activities that involve modified board games and electronic games, such as Battle Ship and Sudoku, projects, such as rubber band/mousetrap cars, and maglev trains, were used to further engage students. It was believed that students would not only see the relevance of algebra in their everyday lives, but also would enhance their computing skills in areas of CAD, illustration, electronic presentation, and spreadsheet software. Students further develop visual skill in using both 2D and 3D graphics as a way of communication, while content understanding is enhanced through developing static and dynamic models.
During the ongoing evaluation process of the Algebra I companion course, the Biology I curriculum for the STEM project began its initial development through the already existing CTE curriculum, called Scientific and Technical Visualization I and II. This second biology based curriculum for the project should require less modification since most of the content already exists within the CTE Scientific and Technical Visualization curricula currently being taught under the technology education scope and sequence. However, expertise is needed to extract the biology content from curricula and add new activities for those areas within the academic course of Biology I that are not represented well in the Scientific and Technical Visualization curriculum. Initial development of this curriculum change took place during the 2008-2009 academic year, with the prospect of piloting this companion course for Biology I during the 2009-2010 academic year.
This STEM-based project must first be accepted by professionals in the fields of CTE and technology education. The researchers would like to see this project expand not only to other states, but also into additional courses in both mathematics and science. Suggested courses for this type of companion course development would include Algebra II, Geometry, Earth and Environmental Science, and Chemistry, just to name a few identified by the research con-ducted within this project (see Figure 1). The authors of this article believe that by including the proposed STEM courses under the area of computational science and including engineer-ing and design, the two skill sets needed for the 21st century as indicated by Murnane and Levy (2004) will have been met.
Mathematics Model | Technology |
---|---|
Algebra I | Virtual & Physical Modeling – Algebra I |
Algebra II | Virtual & Physical Modeling – Algebra II |
Geometry | Virtual & Physical Modeling – Geometry |
Science Models | Technology |
Biology | Scientific Visualization – Biology |
Earth & Environmental Science | Scientific Visualization – Earth & Environmental Science |
Physical Science | Scientific Visualization – Physical Science |
Overall, this is a “win-win” scenario for all involved. Students get a chance to take addition-al courses that further establish relevance of academics while gaining valuable computing skills. Academics get a “boost” within the accountability movement, and teachers perhaps experience less classroom management problems because of heightened student engagement.
In addition, there is the potential for more students to pass their courses and stay in school. More students complete a sequence of CTE courses, increasing its ability to address the drop-out problem plaguing most schools. With the current focus for education on academics, this allows CTE to play an equal role in the education of students. Curriculum development should show that STEM and the integration model proposed nationally can be highly effective, indicating that STEM is not just for the academically gifted students; it can be used to help a significant portion of students understand relevance, accept rigor, and pass end-of-course tests. There are several additional reasons why this project should be implemented at the national level through technology education. The first two are its timeliness and its imperative need. At no time in recent history has there been more concern voiced (by policy leaders, practitioners, and citizens) for acting on the problems that call for high school reform. By developing curricula offered as companion courses to academic courses taught in every high school, schools will not be required to implement major changes in course offerings. However, adopting this project’s strategies will entail a major change in the way science, technology (applied engineering), and mathematics education pro-grams are offered. Also, the project addresses the spirit and intent of the national No Child Left Behind legislation—serving all children well by providing an education that enables them to become responsible, contributing, and participating citizens.
A National Need for Computational Science to be Taught in Technology Education; beyond Engineering and Design
The President’s Information Technology Advisory Committee wrote: “Computational Science—the use of advanced computing capabilities to understand and solve complex problems—has become critical to scientific leader-ship, economic competitiveness, and national security. The membership of the PITAC believe that computational science is one of the most important technical fields of the 21st century because it is essential to advances throughout society” (p. iii). The report continued: “Global competitors are increasingly testing U.S. pre-eminence in advanced R&D and in science and engineering-based industries” (p. 7). Further, the PITAC stated: “We are now at a pivotal point, with generation-long consequences for scientific leadership and economic competitiveness if we fail to act with vision and commitment” (p.18).
The authors of this article are proposing to expand the current technology education program by adding at a minimum one science-based and one mathematics-based course and preferably two science-focused and three mathematical-focused courses. Beyond the argument offered by the PITAC, there are two other courses directly related to CTE’s and technology educators’ mission at the national level. The first argument is predicated on the new Perkins Act and pedagogical theory; the second, on experiences learned while implementing the NC-STEM Project over the past two years.
First, the new Perkins legislation requires CTE to take greater responsibility in helping students understand and apply academic concepts. The companion course structure clearly assists in the application of academic concepts. The pedagogical assumption is that STEM strategies make sense and work. For the purpose of this proposal, a STEM project is defined as the integration of three curricula: science, technology (encompassing engineering at the K-12 level) and mathematics. STEM is essentially an integration strategy. There is ample research evidence indicating curriculum integration is effective, although more difficult to implement at the high school level.
The second argument is more practical and comes from lessons learned through the NC-STEM Project. The initial idea for NC-STEM was to serve a cohort of students who would be concurrently enrolled in math, science, and technology (Scientific and Technical Visualization) courses. Integrated activities would be created which would incorporate concepts and principles from each of the three areas. The idea seemed to make sense, but turned out extremely difficult to put into practice. Therefore, a simpler strategy of pairing two courses together as companion courses, rather than trying to link three courses, made for a more focused approach. Designing companion Scientific and Technical Visualization courses for a particular science such as biology makes it easier to stay focused on the specific essential science ideas. Further, companion courses enable administrators to sequence the course to reflect the sequencing used in science programs.
This same argument was the rationale for the proposal of the Mathematical Modeling and Analysis sequence. However, there is a difference between the science and mathematics computational sequences in that the computational mathematics courses are structured to heavily incorporate physical modeling where the computational science sequence relies primarily on virtual modeling.
While STEM strategies serve both gifted and at-risk students well, the Computational Science Program would permit academically struggling students to apply simple and complex modeling tools to better understand science, technology, engineering, and mathematics concepts and principles. It is expected that the strategies incorporated in this program will make for increased understanding possible for students who would otherwise fail to reach a high degree of technical and academic attainment in traditional settings. The computational science courses are meant to be taken as companion courses, but do not have to be as long as students have the academic area reinforced at some given point.
Conclusions and Recommendations
Reflected in this article are the collective views of the authors as they consider the future of technology education for the next 10 to 20 years. Technology education is yet at another crossroads with its professional interests and associations. Currently, technology educators have embraced engineering and design as core concept. The authors conclude that as long as the concepts taught within the new core areas reflect best practices and technological literacy for all students, from the gifted students to the students at risk for failing, success will (for most students) follow through integration brought about by STEM.
The authors believe that technology education can work in collaboration with engineering groups so that all students can gain from taking a class in technology education. As educators prepare students to be expert thinkers in the 21st century, they must keep in mind that the study of engineering and the overall applied concepts that can come from this area can be appropriate for most students. Further, design processes are also major contributors to students’ understanding of products and sequences. By establishing design as a study within the micro- , human- , and macro-built worlds, students will learn all facets associated with these products and processes and will have a better understanding of the role design plays in several disciplines outside of traditional graphic arts. Design processes can serve as the integrator and driving force behind curriculum development targeting complex communication.
The area of computational science incorporates a truly new way of seeing what technology education can do to support both state and federal initiatives in education. By having courses that link science and mathematics to technology through the development of both virtual and physical models, STEM content integration can take place for students. CTE also is at a cross-roads; the future of CTE may not be the traditional training of more automotive technicians, cabinetry makers, or cosmetologists, but the enhancing and support for academic areas using the established pedagogy that works well with students.
Overall, the future of technology education is yet to be determined and no one can forecast with certainty the course of direction. It is the belief of the authors of this article that provided the current educational climates; technology educators must demonstrate how they can enhance learning of academic areas centered on technological literacy needed for the 21st century.
Note: This paper was presented at the 94th Mississippi Valley Technology Teacher Education Conference in Rosemont, IL
Dr. Aaron C. Clark is an Associate Professor of Technology, Engineering and Design Education at North Carolina State University in Raleigh, and is a member of the Alpha Pi Chapter of Epsilon Pi Tau.
Jeremy V. Ernst is an Assistant Professor in the Department of Mathematics, Science, and Technology Education at North Carolina State University in Raleigh, and is a member of the Gamma Tau Chapter of Epsilon Pi Tau.
References
Brown, B. (2003). The benefits of career and technical education: Trends and issues alert. Office of Education Research and Improvement, Washington, DC. ED 481 326.
Clark, A. C., Wiebe, E. N., Petlick, J., & Ferzli, M. (2004). VisTE: Visualization for technology education; An outreach program for engineering graphics education. Proceedings of American Society for Engineering Education, Southeast Section. Gainesville, FL.
Cushman, K. (1989). Asking the essential questions: Curriculum development. Horace, 5(5). 17-24.
Gomez, A. G. (2001). History and philosophy of technology education: Technology education time-line university of wisconsin-stout. Retrieved December 28, 2008, from http://www.imagine101.com/title.htm
Hevesi, A. G. (March 2, 1999; October 16, 2007). Schools lack math and science needs. New York Times.
International Technology Education Association (ITEA, 2005). Technology for all americans project. Retrieved October 16, 2007, from http://www.iteaconnect.org/TAA/ TAA.html
Murnane, R. J. & Levy, F. S. (2004). The new division of labor: How computers are creating the next job market. Princeton, NJ: Princeton University Press.
National Association of Gifted Children (NAGC, 2008). Glossary of gifted terms. Retrieved December 27, 2008, from http://nagc.org
National Coordination Office for Networking and Information Technology Research and Development (2007). Your federal networking and it r&d resource. Retrieved October 15, 2007, from http://www.nitrd.gov/
Partnership for 21st Century Skills (2004). U.S. students need 21st century skills to compete in a global economy. Retrieved October 15, 2007 from http://www.21stcenturyskills.org/index.php
President’s Information Technology Advisory Committee (PITAC, 2005). Report to the President on computational science: Ensuring America’s competitiveness. Washington, DC: U.S. Government Printing Office.
Public Schools of North Carolina (2007). Accountability services. Retrieved October 15, 2007, from http://www.ncpublicschools.org/accountability/
Reddick, L. A., Jacobson, W., Linse, A., & Yong, D. (2007). An inclusive teaching framework for science, technology, engineering, and math. In M. Ouellett (Ed.), Teaching inclusively: Diversity and faculty development. Stillwater, OK: New Forums Press.
Stone, J. R. & Alfeld, C. (2004). Keeping kids in school: The power of CTE. Techniques, 18(3), 28-29.
U. S. Department of Education (2007a). Reauthorization of no child left behind. Retrieved October 15, 2007, from http://www.ed.gov/nclb/landing.jhtml
U. S. Department of Education (2007b). Office of vocational and adult education. Retrieved October 15, 2007, from http://www.ed.gov/about/offices/list/ovae/index.html?src=mr
Varnado, T. E., & Pendleton, L. K. (2004). Technology education/engineering education: A call for collaboration. Proceedings of the International Conference on Engineering Education. Gainesville, FL.
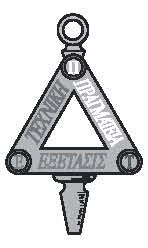
TS