JOTS v37n2 - Sustainable Design: An Educational Imperative
Sustainable Design: An Educational Imperative
David Klein and Ken Phillips
Abstract
This contribution presents a curriculum model and pedagogy for teaching sustainability concepts to industrial design students at the Metropolitan State College of Denver (MSCD). The curriculum provides students with instruction about low-impact material and process selection (renewable, toxic, embodied energy); energy efficiency (transportation, processing, use of product); quality and durability; design for reuse/recycling; and social relevancy/value, consumer value, responsible production/procurement (fair labor). Although the authors are aware of the interrelationship of these categories, they isolated each one to make them easier to teach, explain, and assess.
In our industrial design studio courses, sustainability and product life cycle assessment are established as design parameters for all projects throughout the curriculum. Sustainable principles are internalized as students devise practical design solutions. This pedagogy enables students to practice sustainability in relation to product design as they conduct product feasibility studies, design development, and production planning.
This article aims to advance design education by serving as an example of how sustainability may be taught and integrated into a curriculum and by providing case studies of completed student design projects.
Introduction
Design is concerned with the development of products, tools, machines, artifacts, and other devices produced on a massive scale, resulting in a profound and direct influence on ecology (Papanek, 2003). When design students are made aware of the context in which products are manufactured, they are more likely to consider barriers to sustainability outside of their usual parameters; increasingly resource-hungry technology, a culture that focuses on the short term, and pervasive advertising and marketing efforts aimed at increasing consumption. According to Orr (1994) , “the truth is that without significant precautions, education can equip people merely to be more effective vandals of the Earth” (p. 5).
The traditional definition of sustainability calls for policies and strategies that meet society’s present needs without compromising the ability of future generations to meet their own needs. Industrial designers make their living developing designs that meet the needs of the present, but are not always bound to consider future generations. They are integral components of a manufacturing system that maintains product flow, and they make decisions concerning the production processes and materials that, in turn, have the most impact on whether or not a product is sustainable. It is particularly important that industrial designers be aware of—or even be—champions for sustainable practices. Principles of sustainability can stimulate technological innovation, advance competitiveness, and improve quality of life. Sustainable development reflects not only the trade-off between business and the environment but also the synergy between them. A responsible design educational program should help students realize that environmental protection does not preclude economic development and economic development must be ecologically viable now and in the long run.
True sustainability involves a wider range of issues than the design, manufacture, and disposal of industrially produced products. The numerous factors that contribute to a culture of consumption and consumerism beg to be considered, “ . . . the urge to consume is merely symptomatic of a stimulus-hungry species dwelling in a homogenized and over-streamlined world where the prevailing mode of existence comes with the majority of problems already solved” ( Chapman, 2005 , p. 29). Designs embedded with meaning, those that stimulate consumers on a multitude of levels (functional, emotional, status reinforcement, etc.), are less likely to be victims of product replacement ( Chapman, 2005 ). Notable exceptions to this concept are "technology" objects, like computers, whose existence relies on software and microchips that apparently require an update every two years, according to some. Our Industrial Design program has developed a curriculum that addresses the following categories of sustainability concepts, listed in no particular order of relevance.
- Low-impact, renewable, nontoxic material selection, minimal embodied energy requirements
- Efficiency in transportation, consumer use, and manufacturing of products
- Life cycle assessment
- Product design that considers reuse/recycle
- Social relevancy of concept/product.
This article presents the pedagogy and curriculum utilized in teaching these sustainability concepts to industrial design students at MSCD.
Sustainability Issues and Industrial Design
The sum total effect of factors contributing to ecological impact of a product, or a society, may be cumulatively referred to as the "ecological footprint" that is "occupied." Plato may have been the first to acknowledge an ecological footprint when he realized that adequate human support cannot be fixed without consideration of the land and surrounding resources. Modern ecological footprint analysis is an accounting tool that enables us to estimate the resource consumption and waste assimilation requirements of a defined human population or economy in terms of a corresponding productive land area ( Wackernagel & Rees, 1996 ).
It is especially important to recognize that economic and ecologic sustainability go hand in hand. There may, in fact, be capitalist motivations that spearhead efforts toward true long-term sustainability. Smart entrepreneurs, movers, and shakers invest their time and capital in endeavors that offer economic growth and prosperity, and long-term commercial success relies on acceptable environmental performance. Excessive resource depletion and pollution are disruptive and costly, and it can call into question the social responsibility of a company ( Mackenzie, 1997 ). People are accustomed to thinking of industry and the environment as being at odds, because conventional methods of extraction, manufacture, and disposal have historically been destructive to the natural world. It is a condition that complicates efforts to achieve sustainability. "As natural as drawing breath, the urge to consume is merely symptomatic of a stimulus-hungry species dwelling in a homogenized world where the prevailing mode of existence comes with the majority of problems already solved" ( Chapman, 2005 , p. 29). But our environment is saturated with messages and images to remind us that we, indeed, need more. Studies have shown that the more people look outside of themselves as a way of satisfying needs (affluenza, peer approval, etc.), the less likely they are to have their actual needs met ( Thorpe, 2007 ). The "environmental crisis" is perhaps less an environmental and technical problem than it is a behavioral and social one ( Wackernagel & Rees, 1996 ). But there is a need for playfulness and desire in our lives which consumer-led design helps to meet. It is the extent to which these products have become dominant that is troublesome. Papanek (1973) held that “in an age of mass-production when everything must be planned and designed, design has become the most powerful tool with which man shapes his tools and environment (and by extension, society and himself)” (p. 14).
Higher education has largely been shaped by the drive to extend human domination, to "manage" planet Earth to its fullest. Design competence requires the integration of first-hand experience and practical competence with theoretical knowledge ( Orr, 1994 ). Our leaders and technologists have been educated in a system that emphasizes theories instead of values, concepts rather then human beings, abstraction rather then consciousness, answers instead of questions, efficiency rather than conscience. This points to the fact that education itself is no guarantee of decency or wisdom. "It is not education, but that of a certain kind that will save us" ( Orr, 1994 , p. 8). Designers have not been taught in consideration of the larger social context and ecological impact, so they assume that their area of responsibility is limited to functional and aesthetic experiences ( Mackenzie, 1997 ). The value of contributing to positive social change emphasized by Papanek (2003) and others has more recently been downplayed in favor of form giving and branding. “The action of the profession has been comparable to what would happen if all medical doctors were to forsake general practice and surgery and concentrate exclusively on plastic surgery and cosmetics” ( Whiteley, 1993 , p. 99). In the end “the most important ability that a designer can bring to their work is the ability to recognize, isolate, define and solve problems” ( Papanek, 1973 , p. 160).
Design approaches that embrace the natural world are perhaps more inherently sustainable. The branch of industrial design known as biomimicry begins with an examination of natural systems, particularly the many biomechanical characteristics found in nature. The rationale for this approach is the ideas that appropriate solutions for a given natural life form may provide inspiration for "natural" solutions to man-made problems ( Birkeland, 2002 ). It also reinforces respect for nature and serves as a reminder that we are "of " nature, and not simply existing within it. Biomimics explore what works, and what lasts, in the natural world.
The more our world looks and functions like the natural world, the more likely we are to exist in harmony ( Benyus, 2002 ). Natural forms and patterns famously inspired design styles like Art Nouveau, but there is more to learn by studying the deeper underlying principles found in nature. Examples of biomechanical principles applied to product design include pliers based on the mechanics of the human jaw, Velcro based on the Burdock plant cockleburs, ball-and-socket joints based on the human hip joint, tensile fibers inspired by spiderwebs, and a drinking fountain that mimics a leaf form ( Klein, 2010 , Figs. 1 & 2). Designers can benefit in many ways by being students of the natural world.
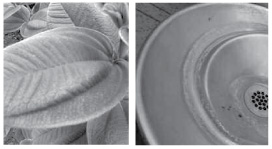
Case Studies
Designs that show particular sensitivity to each category of sustainability in our curriculum may provide further understanding of the underlying principles.
Wood-based materials utilized for extruded shapes ( Guidot, 2006 , p. 123, Fig. 3) are a renewable material alternative to plastic and metal, while specifying aluminum alloys with low melting points (Rocky Mounts bike rack, photo by David Klein, 2010 , Fig. 4. Reproduced with permission) reduces manufacturing energy in comparison to other metals. Using recycled aluminum reduces energy required in the bauxite mining process, although it also presents its own energy requirements. Industrial Designer Ali Tayar designed a chair that relies on a single extruded shape, placed in different positions in order to reduce the manufacturing and energy costs associated with multiple tooling dies ( Terragni, 2002 , p. 357, Fig. 5).
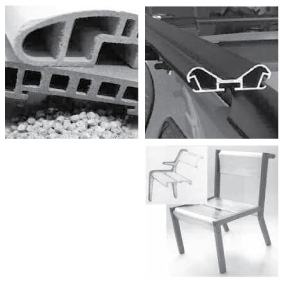
Flat-pack designs conserve shipping space for improved efficiency in transportation. They often result in less material waste if cut shapes can be nestled (Coat rack by Blu Dot, www.bludot.com, Fig. 6. Reprinted with permission). The most effective steps toward efficient manufacturing are simplification of the design and reduction in the number of required components ( Bralla, 1999 ). This idea is illustrated in a nail clipper design comparison by Professor Karl Ulrich, of the Wharton School ( Bralla, 1999 , p. 7.9, Fig. 7. Reprinted with permission). The recognizable design on the bottom is improved in respect to efficiency, functionality, and aesthetics.
The Heat Wave electric radiator for Droog by Joris Laarman, (Photographer: Robaard/Theuwkens, Styling by Marjo Kranenborg, CMK, Fig. 8. Reprinted with permission) offers increased surface for airflow opportunity so that more heat is obtained with less energy than the traditional design (public domain, Fig. 9). A more interesting aesthetic is also achieved, and less floor space is required.
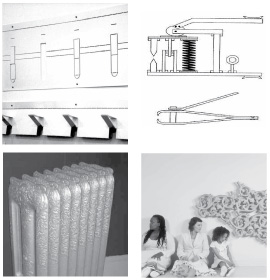
Life cycle assessment of plastic cutlery reveals a life span that far exceeds functional requirements. Cutlery made from plant starch (www.ecoproducts.com, Fig. 10. Reprinted with permission) or semolina flour material (Designer unknown, Fig. 11) offers a sustainable alternative to petroleum-based plastic utensils and will decay in landfills more rapidly.
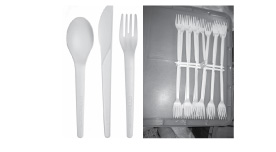
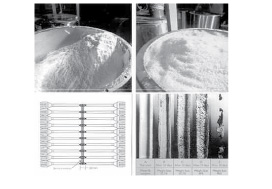
The Heineken WOBO (short for WOrld BOttle) bottle never made it to the consumer market ( Terragni, 2002 , p. 418, Fig. 12). The concept of this bottle considered product reuse as a building "brick." The bottles were designed to fit modularly, with dimples on the flat surfaces to grip mortar.
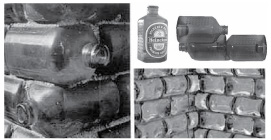
The Hanger design offers an opportunity to reuse plastic bottles (Design by Xuan Yu , for the RE-think + Re-cycle competition at www.designboom.com, Fig. 13. Reprinted with permission). Even though such designs may not win wide public acceptance initially, it is more important that they provide inspiration for future designs that will.
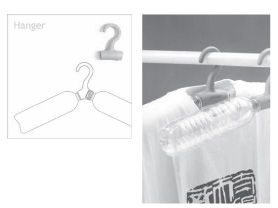
The Mirra chair, produced by Herman Miller, represents an admirable model of sustainably produced furniture. The production line uses 100% green power (50% wind turbines, 50% captured off-gassing from landfills), and 96% of Mirra's content is specifically designed for recycle or reuse. The exceptions follow (courtesy of Herman Miller Inc., Fig. 14. Reprinted with permission).
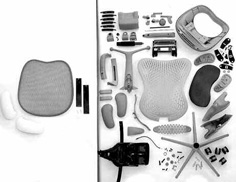
The $50 wheelchair (actually $51.29) was designed by mechanical engineer Don Schoendorfer in 1999 and is distributed by his Free Wheelchair Mission (www.freewheelchairmission.org, Fig. 15). It utilizes many existing components in order to minimize tooling costs, and it helps to sustain the livelihood of people who otherwise would literally have to crawl from place to place.
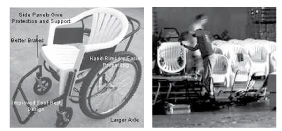
The Tree bookshelf designed by Shawn Koh (www.designartist.co.kr, Fig. 16) reinforces the relationship between trees and books (paper comes from trees), encourages diverse reading through the use of diverse “shelf' shapes,” and inspires by making it fun to interact with books. Each of these designs illustrates social relevancy that can be achieved in designed, manufactured products.
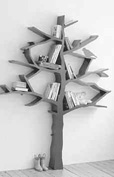
Pedagogy at MSCD
Sustainability and product life cycle assessment are among the design parameters for studio design projects. The principles are internalized as students devise practical design solutions that draw from myriad sources. This pedagogy requires students to consider and employ sustainability concurrently with product design/development, as opposed to a curriculum in which sustainability is taught isolated, in lecture courses. But we do offer specific information in an instructional format to facilitate students developing a background in the application of sustainability principles. These techniques include lectures and presentations about contemporary and historical models of sustainable design thinking; the use of required readings in the content area; discussions; workshops and presentations by outside experts; and the application of this information.
The goal of this instructional program is to enable students to make informed design decisions regarding sustainability issues for their product designs. In addition to this comprehensive goal, the department faculty has developed specific learning objectives. Upon completion of the Industrial Design program, major students should be able to:
- Recall, discuss, and apply information about low-impact materials—renewable materials, awareness of material toxicity, and the relative embodied energy of materials.
- Evaluate and choose design solutions that provide increased energy efficiency in transportation, consumer use of products, and manufacturing processes.
- Identify and discuss components of life cycle assessment techniques and select design solutions for maximal product quality and durability.
- Design products that facilitate their reuse or recycling at the end of their lifecycle.
- Select product designs, materials, and processes that have a positive social impact.
Assessment is an important element of any instructional program. The evaluation of students in regards to the objectives listed above occurs in several ways. There is an embedded component in the evaluation/critique of student designs by faculty and professionals that occurs in studio classes. Sustainability concepts tied to the instructional materials are part of the overall assessment of student designs. There are embedded elements of assessment for these objectives in quizzes and tests for some courses where the specific instruction occurs, and an overarching component to the assessment of these objectives. The capstone design studio course and the senior internship are evaluated for a number of student performance categories reflecting sustainability objectives and overall learning objectives for students so they will be able to perform the practice of industrial design. This assessment is administered both by faculty and practicing professionals, and it provides key data for the ongoing evaluation of the program. These results direct faculty so they can continue to improve both curriculum and instructional practices so they will reflect the assessment data. The faculty believe that evaluating department students’ ability to utilize informed sustainable design decisions should be addressed at the more global outcome level; this would allow for an assessment of the integrated whole of the lower level courses and objectives regarding sustainable design. Program-level assessment data regarding sustainable design is discussed in a later section of this article.
The Curriculum Model
Although the authors are aware of the interrelationship of the categories represented in the learning objectives, their curriculum isolates each one to make them easier to teach, explain, and assess. Working definitions of the topics included in the categories follow.
1. Low-impact renewable, nontoxic material selection with minimal embodied energy requirements.
The working definition in the curriculum describes low-impact renewable materials as those that can be derived from agricultural crops (grown for nonfood uses) and from other plant or animal sources. They are renewable because they can be used and replaced without irreversibly depleting reserves; this property also makes them a valuable resource in combating climate change. Nontoxic material considerations delineated in coursework are based on the premise that "toxicity" refers to a material's ability to harm living things and at what level of exposure ( White, St. Pierre, & Belletire, 2007 , p. 17), Issues of concern for designers in relation to product consumer use addressed in the curriculum are: out-gassing issues, the use of carcinogens-materials considered capable of causing cancer, mutagens or materials that induce genetic changes in the DNA of chromosomes, and teratogens, a material or agent that causes physical defects in a developing embryo (www.epa.gov/enviro/html/emci/chemref). An awareness of research methods into material toxicity issues, the use of material safety data sheets, and material toxicity concerns in the manufacturing process are also addressed.
As presented in course lectures, embodied energy refers to the quantity of energy required to manufacture, and supply to the point of use, a product, material, or service. Embodied energy considers the energy requirements for materials from the raw material extraction, to transport, manufacturing, assembly, to produce a service or product, and finally to bring about its disassembly, deconstruction and/or decomposition. ( White, St. Pierre, & Belletire, 2007 ).
2. Efficiency in transportation, consumer use, and manufacturing of products.
Transportation issues are discussed and emphasized, including the use of flat-pack designs and ready-to-assemble products. Packaging for shipping container efficiency, and product weight considerations are addressed. Energy considerations in regard to the consumer use of products are addressed in design discussions. Possible design solutions that utilize hand-power options, low-energy-consuming bulbs, auto shut-off features, and others are encouraged.
Manufacturing processes are important topics in the curriculum because they require so much energy, comprising as much as 80% of industrial energy use. Manufacturing plays a big role in U.S. energy use. Industry accounts for around 30% of the total, and manufacturing is responsible for around 80% of industrial use ( Halber, 2006 ). The ability to research the energy requirements for different manufacturing processes is developed through the coursework.
3. Life-cycle assessment (LCA)
A critical challenge for designers is to embrace the “material trail” of their work. Awareness of the true amount, type, and source of materials behind their designs is a good step toward understanding the challenges of ecological sustainability ( Thorpe, 2007 ). The question, "Paper or plastic?" posed by the grocery store clerk, actually opens up myriad questions and concerns that experts are continuing to debate over. In the end, it is best to bring a reusable bag, and answer "neither."
Because of the multiple factors and complexities in making decisions about design based on LCA, there are most often no clear “correct” answers. Challenges and opportunities may be realized in comprehensive product life-cycle assessments, but at the undergraduate level such complex assessments are difficult to construct and evaluate. It is the authors’ aim to introduce the guiding concepts behind such detailed analysis and make students aware of the complexities that contribute to truly sustainable industrial design.
4. Product design that considers reuse/recycle
An assumption that guides the curriculum in this category is that a potential route to an environmentally friendly design solution is to extend product life through designs that allow repair and reuse. When the product is no longer usable, the materials and components can be recovered through recycling. Reuse is the recovery of materials and/or components for similar or alternate end use. Recycling turns materials that would otherwise become waste into resources for remanufacture in new products.
Authors of Cradle to Cradle ( McDonough & Braungart, 2002 ) have referred to recycling as down cycling, as it reduces the quality of a material over time (and increasingly over multiple recycles). In some cases using materials that were never designed for their new application, reprocessing them into a new form, may require as much energy—and generate as much waste—as employing virgin materials, but without reliable, known characteristics ( McDonough & Braungart, 2002 ). The trend in U.S. industry, and the U.S. EPA, is toward preventing wastes before they are created instead of treating, disposing, or reprocessing them later ( Ciambrone, 1997 ). This is an indication that currently in industry many are realizing that the impact their products have on the environment is not limited to the manufacture of a product.
5. Social relevancy of concept/product
It is good for designs to be thematically pointed toward solutions that have positive impact on people and society. Designers must work to replace semantically loaded expressions as “beautiful,” “ugly,” “cool,” “cute,” and so on, with words like “meaningful” ( Papanek, 1973 , p. 25). It is important for ecologically intelligent products to be meaningful and to be at the forefront of human expression ( McDonough & Braungart, 2002 ). Many examples of new technology and devices have languished because they were ahead of their time, including pneumatic tires, the laser, and fluorescent light bulbs ( Volti, 2006 ). It is not enough for sustainable solutions to simply exist. There must be a desire for them. Their end should elegantly glorify their means.
Specific Curriculum
Currently, multiple courses deal with the topics regarding the above sustainable design considerations. In analyzing the instructional coverage of these topics the department has found it beneficial to chart the courses that address each topic, and to what extent. A matrix was developed that indicates where the topics are introduced (I), where they are reviewed and reinforced (R), and where the students demonstrate (D) application of the concepts. While the specific pedagogy may vary from faculty to faculty as course assignments change, the matrix representing these instructional steps remains in place. Assessment results may indicate the need for change over time, but Table 1 illustrates the current curricular plan.
Sustainable Design Topics in Required Courses | Materials Classes | Manufacturing Materials and Processes Class | Beginning ID Studio | Intermediate ID Studio | Design for Production | Advanced ID Studio | Professional ID Studio | Concept and Portfolio Design | Professional internship |
---|---|---|---|---|---|---|---|---|---|
I= Introduced
R= Reviewed and reinforced D= Demonstrates application/integration in design solutions |
|||||||||
Renewable materials | I | R | R | R | R | R | D | D | |
Materials toxicity | I | R | R | R | R | D | D | ||
Embodied energy | I | R | R | R | R | D | D | ||
Transport efficiency | I | R | R | D | D | ||||
Consumer energy use | I | R | R | D | D | ||||
Efficient manufacturing processes | I | R | R | R | R | D | D | ||
Life cycle assessment | I | R | R | D | D | ||||
Re-use/recycle | I | R | R | R | R | R | D | D | |
Positive social impact | I | R | R | D | R | D |
Examples of how we address a specific sustainable design topic through the curriculum
Information about renewable materials and associated considerations are introduced initially in the materials courses. Students are made aware of the renewable nature of certain materials and are given information on available “green” materials. For example, in the department’s Introductory Woodworking classes students are introduced to the industrial materials available for wood-based designs as well as how to price, specify, and order those materials. As a part of these presentations the use of green materials, such as FSC certified lumber and alternative quick growing resource sheet materials (bamboo and Kirei sorghumbased board), and sources for recycled lumber are relayed to the students. These considerations of renewable material use are reviewed and reinforced as they pertain to manufacturing processes in the department’s manufacturing materials and process class. The same concepts are addressed with prompting from instructors as students address design problems in the lower level studio sequence classes and also the design for production class in regards to material selection. Students are expected to demonstrate an ability to apply renewable material considerations in the capstone studio class and during their internship placements.
Program Assessment of Sustainable Design
Our department believes that sustainable design should be assessed at the more global, program outcome level. Assessing them at the course level downplays the value of true integration of sustainability into the wider pedagogy and curriculum. We seek to integrate rather than separate this important subject matter. This section reports on the program assessment results regarding sustainable design. The Industrial Design program utilizes three different data-collection methods, which are tied directly to the program’s student learning outcomes, and it provides evaluators with an opportunity to provide input on all of them. The data-collection instruments are utilized by internship supervisors, faculty, and professionals observing senior presentations; student interns use it for self-evaluations regarding how well the program has prepared them. The three instruments evaluate students at or near the end of the program and thus can assess the integrated nature of the sustainability outcome. Although there are seven overall program-level learning outcomes, the instruments expand those outcomes into 22 elements for an improved ability to address specifics. Sustainable design is one of the 22 elements that are assessed each year as part of the regular program assessment process.
The department has instituted a minimum target score of 80% (or 3.2 on the 4-point scale) on the evaluation instruments. This is consistent with faculty expectations of meeting standards to succeed in the profession and represents what the faculty believe is an appropriate expectation for student achievement. The current data-collection instruments have been in place for two years. Though this report will include comments comparing this year’s scores to last year’s data, it is probably premature to identify clear trends from this limited sample. Figure 17 shows the current level of achievement regarding student knowledge and application of sustainable design concepts. The data show a trend toward improved scores for student achievement, over the relatively short time span of two assessment cycles.
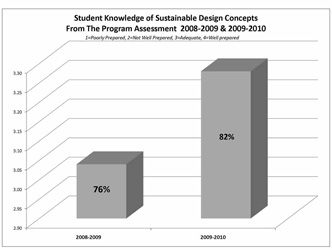
Conclusions
An academic institution’s approach to teaching sustainability will vary widely depending on the clientele that it serves and the context in which the institution exists. Given our program’s context and the nature of our student body, the most appropriate focus for our program is on the material, technical aspects of ecological solutions that may be readily applied. The department exhibits a commitment to the concept of sustainable design with ongoing work to educate students and encourage appropriate sustainable design solutions. The ongoing program assessment data will direct instructional and curricular adjustments to improve the current pedagogical strategies for this vital design imperative.
*This article is based in part on a conference paper presented at the NCIIA 13th Annual Meeting on March 19-21, 2009. Washington DC.
Dr. David Klein is an Associate Professor of Industrial Design at Metropolitan State College of Denver, Colorado. He is a member of the Nu Field Chapter of Epsilon Phi Tau
Dr. Kenneth R. Phillips is the Department Chair for the Industrial Design Department at Metropolitan State College of Denver (MSCD).
References
Benyus, J. M. (2002). Biomimicry: Innovation inspired by nature . New York: Harper Perennial (reissue).
Birkeland, J. (2002). Design for sustainability: A sourcebook of integrated, eco-logical solutions . London: Earthscan Publications Ltd.
Bralla, J. G. (1999). Design for manufacturability handbook . New York: McGraw-Hill.
Chapman, J. (2005). Emotionally durable design . London: Earthscan.
Ciambrone, D. F. (1997). Environmental life cycle analysis . New York: Lewis Publishers. Retrieved from http://www.epa.gov/Sustainability/basicinfo.htm
Guidot, R. (2006). Industrial design techniques and materials . Milan: Flammarion.
Halber, D. (2006). Engineer launches review of energy use in manufacturing . Retrieved from http://web.mit.edu/newsoffice/2006/manufacturing-1101.html
Klein, D. W. (2010). Original photos of figure 1 (Rocky Mounts bike rack, www.yakima.com, reprinted with permission), and figure 2 (water fountain, designer unknown).
Mackenzie, D. (1997). Green design: Design for the environment . London: Laurence King.
McDonough, W., & Braungart, M. (2002). Cradle to cradle: Remaking the way we make things . New York: North Point Press.
Orr, D. W. (1994). Earth in mind: On education, environment, and the human prospect . Washington: Island Press.
Papanek, V. (1973). Design for the real world: Human ecology and social change . Toronto: Bantam Books.
Papanek, V. (1995). The green imperative: Ecology and ethics in design and architecture . London: Thames & Hudson.
Thorpe, A. (2007). The designer's atlas of sustainability: Charting the conceptual landscape through economy, ecology, and culture . Washington: Island Press.
Terragni, E. (Ed.). (2002). Spoon, 100 designers . Phaidon Press Limited.
United States Environmental Protection Agency (EPDA). (2011). What is sustainability? Retrieved from http://www.epa.gov/sustainability/basicinfo.htm#sustainability
Volti, R. (2006). Society and technological change (5th ed.). New York: Worth Publishers.
Wackernagel, M., & Rees, W. (1996). Our ecological footprint, reducing human impact on the earth . Gabriola Island, BC: New Society Publishers.
White, P., St. Pierre, L., & Belletire, S. (2007). Okala course guide . Dulles, VA: IDSA.
Whiteley, N. (1993). Design for society . London: Reakton Books LTD.